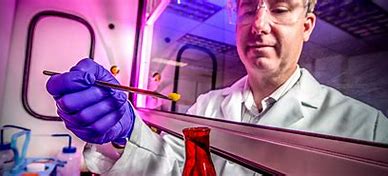
Introduction
Explosives, with their immense power and destructive potential, have captivated the human imagination for centuries. From military applications to industrial uses and criminal activities, explosives play a significant role in various aspects of modern society. However, understanding the nature of explosives and effectively analyzing them is essential for mitigating risks, ensuring public safety, and investigating incidents involving their use. In this comprehensive blog, we embark on a journey to explore the fascinating world of explosives, their types, properties, and the intricate methodologies employed in their analysis.
Understanding Explosives:
Explosives are substances capable of undergoing rapid and violent decomposition, resulting in the release of energy in the form of heat, light, sound, and pressure. They are classified into two main categories: high explosives and low explosives. High explosives, such as dynamite and C-4, detonate with supersonic shock waves, while low explosives, such as black powder and gunpowder, deflagrate at subsonic speeds. Explosives can further be categorized based on their chemical composition, sensitivity to initiation, and intended use.
Types of Explosives:
Nitrogen-Based Explosives: Nitrogen-containing compounds, such as nitroglycerin and TNT (trinitrotoluene), are among the most commonly encountered explosives. These compounds release energy through the rapid oxidation of nitrogen during detonation, producing high-pressure gases and heat.
Organic Peroxides: Organic peroxides, such as acetone peroxide and triacetone triperoxide (TATP), are highly unstable compounds formed by the reaction of organic solvents with hydrogen peroxide. They are often used in improvised explosive devices (IEDs) due to their ease of synthesis and availability of precursors.
Ammonium Nitrate: Ammonium nitrate, a widely used fertilizer, can also serve as an explosive when combined with a fuel source, such as diesel fuel or aluminum powder. The combination forms an improvised explosive mixture known as ammonium nitrate-fuel oil (ANFO), which has been used in numerous terrorist attacks and industrial accidents.
Military Explosives: Military explosives, such as RDX (cyclotrimethylenetrinitramine) and PETN (pentaerythritol tetranitrate), are specifically designed for military applications, including demolition, munitions, and ordnance.
Advanced types of explosives:
Recent advancements in explosives have primarily focused on improving efficiency, stability, and concealment, as well as developing novel formulations for specific applications. Some of the advanced types of explosives include:
Insensitive High Explosives (IHEs):
Insensitive high explosives are designed to be less sensitive to external stimuli such as impact, friction, and heat, while retaining their explosive power. These explosives offer improved safety during handling, storage, and transportation, reducing the risk of accidental detonation. Examples of IHEs include CL-20 (Hexanitrohexaazaisowurtzitane) and FOX-7 (1,1-diamino-2,2-dinitroethylene).
Nanoenergetic Materials:
Nanoenergetic materials are a class of energetic materials that harness the high surface area and reactivity of nanomaterials to achieve enhanced performance. Nanoenergetics exhibit rapid energy release, high power density, and tunable properties, making them suitable for various applications, including propulsion, pyrotechnics, and explosives. Examples include nanoaluminum-based energetic composites and nanostructured energetic materials incorporating metal oxides or carbon nanotubes.
Green Explosives:
Green explosives, also known as environmentally friendly explosives, are designed to minimize environmental impact and reduce toxic byproducts during detonation. These explosives utilize alternative oxidizers and fuels derived from renewable or non-toxic sources, such as ammonium dinitramide (ADN) as an oxidizer and sugar-based compounds as fuels. Green explosives aim to mitigate pollution and environmental damage associated with traditional explosives manufacturing and disposal.
Composite Modified Explosives:
Composite modified explosives are engineered by incorporating additives or modifiers into conventional explosive formulations to enhance performance, stability, and safety. These modifiers can improve detonation properties, reduce sensitivity to external stimuli, and enhance compatibility with other materials. Examples include polymer-bonded explosives (PBXs), plastic-bonded explosives (PLXs), and reactive materials embedded within a matrix of an inert polymer or elastomer.
Metastable Interstitial Composite (MIC) Explosives:
Metastable interstitial composite (MIC) explosives are a class of energetic materials that exploit the metastable properties of interstitial compounds to achieve high energy density and rapid energy release. MIC explosives typically consist of a metal fuel, such as aluminum or magnesium, dispersed within a matrix of interstitial compounds, such as hydrides or nitrides. The interaction between the metal and interstitial compounds results in exothermic reactions, leading to explosive decomposition
Non-Detonable Energetic Materials:
Non-detonable energetic materials are designed to possess high energy content without the ability to undergo self-sustaining detonation. These materials are engineered to release energy through deflagration rather than detonation, making them safer to handle and transport. Non-detonable energetic materials find applications in pyrotechnics, propellants, and blasting agents where controlled energy release is desired without the risk of accidental detonation.
Metamaterial-Based Explosives
Metamaterial-based explosives are a recent area of research that explores the use of engineered metamaterials to control and manipulate shock waves produced during detonation. By tailoring the structure and properties of metamaterials at the micro- and nano-scale, researchers aim to enhance shock wave propagation, energy absorption, and blast mitigation capabilities. Metamaterial-based explosives hold potential for improving blast resistance in military and civilian applications.
Analytical Techniques in Explosive Analysis:
The analysis of explosives requires a multidisciplinary approach, incorporating various analytical techniques to identify, characterize, and quantify explosive residues and compounds.
The preliminary and confirmatory examination of explosives is a critical process conducted by forensic scientists and law enforcement agencies to identify, characterize, and analyze explosive materials. This comprehensive examination involves a series of systematic steps aimed at gathering evidence, conducting initial assessments, and performing detailed analyses to confirm the presence of explosives and determine their composition. Below, I outline the key steps involved in both the preliminary and confirmatory examination of explosives:
Preliminary Examination:
Scene Assessment:
Forensic investigators assess the scene of the explosion to document the extent of damage, blast patterns, and potential sources of evidence. They identify and collect samples of suspected explosive residues, debris, and fragments for further analysis.
Visual Inspection:
Forensic examiners visually inspect the collected samples for characteristics indicative of explosive materials, such as color, texture, odor, and crystalline structure. They look for common signs of explosive residues, including soot, charring, pitting, and discoloration.
Screening Tests:
Screening tests, such as color spot tests and chemical reagent tests, are performed to detect the presence of explosives based on their chemical properties and reactions. These tests provide rapid preliminary results to guide further analysis and investigation.
Portable Instrumentation:
Handheld instruments, such as X-ray fluorescence (XRF) spectrometers and ion mobility spectrometers (IMS), may be used for on-site analysis to identify elemental composition and volatile compounds associated with explosives.
Confirmatory Examination:
Sample Preparation:
Collected samples are prepared for confirmatory analysis by grinding, sieving, or homogenizing to ensure representative and uniform samples for testing.
Non-destructive techniques, such as Fourier-transform infrared (FTIR) spectroscopy and Raman spectroscopy, may be used for preliminary identification without sample preparation.
Instrumental Analysis:
Advanced analytical techniques, including chromatography, mass spectrometry, and spectroscopy, are employed for confirmatory analysis of explosives.
Gas chromatography-mass spectrometry (GC-MS) is widely used to separate and identify explosive compounds based on their retention times and mass spectra.
Fourier-transform infrared (FTIR) spectroscopy and Raman spectroscopy provide molecular fingerprinting of explosive residues, allowing for precise identification and characterization.
Microscopic Examination:
Microscopic techniques, such as scanning electron microscopy (SEM) and optical microscopy, are utilized to examine the morphology, size, and distribution of explosive particles and residues.
SEM-EDS (energy-dispersive X-ray spectroscopy) analysis provides elemental mapping and chemical composition of explosive residues at the microscale.
Reference Standards and Libraries:
Forensic laboratories maintain reference standards and libraries containing spectral data, chromatographic profiles, and chemical properties of known explosives for comparison and identification purposes.
Comparison of experimental results with reference standards and databases aids in the confirmation of explosive compounds and formulation of forensic conclusions.
Quality Assurance and Reporting:
Quality assurance procedures, including calibration, validation, and proficiency testing, are implemented to ensure the accuracy, reliability, and reproducibility of analytical results.
Forensic scientists prepare detailed reports documenting the methods, findings, and conclusions of the explosive examination, which may be used as evidence in legal proceedings.
Challenges and Considerations in Explosive Analysis:
Analyzing explosives presents numerous challenges and considerations due to the complexity and hazardous nature of these materials. Some of the key challenges include:
Sample Collection: Collecting representative samples from the scene of an explosion can be challenging due to contamination, environmental factors, and safety concerns. Proper sample handling and preservation are essential to ensure the integrity of the evidence.
Sample Complexity: Explosive residues may contain a complex mixture of compounds, including primary and secondary explosives, degradation products, and environmental contaminants. Analyzing these mixtures requires sophisticated analytical techniques and expertise.
Instrumentation and Method Development: Developing analytical methods for explosive analysis requires specialized instrumentation and expertise. Optimization of parameters such as chromatographic conditions, mass spectrometry parameters, and sample preparation techniques is essential to achieve accurate and reliable results.
Safety Considerations: Working with explosives presents inherent safety risks, including the potential for accidental detonation, exposure to toxic compounds, and environmental hazards. Proper safety protocols and training are essential to mitigate these risks and ensure the safety of laboratory personnel.
Advancements and Innovations in Explosive Analysis:
Advancements in technology and methodology continue to drive innovation in the field of explosive analysis, enabling forensic scientists and law enforcement agencies to overcome challenges and enhance their capabilities. Some notable advancements and innovations include:
Portable Analytical Instruments: The development of portable analytical instruments, such as handheld GC-MS systems and portable X-ray fluorescence (XRF) spectrometers, allows for rapid on-site analysis of explosive residues, reducing the need for sample transportation and expediting the investigative process.
Advanced Spectroscopic Techniques: Advances in spectroscopic techniques, such as hyperspectral imaging and terahertz spectroscopy, offer new opportunities for the detection and characterization of explosive materials. These techniques provide high-resolution imaging and spectroscopic data, allowing for the identification of concealed explosives and trace residues.
Computational Modeling and Simulation: Computational modeling and simulation techniques play an increasingly important role in explosive analysis, enabling researchers to predict the behavior of explosives under different conditions and environments. These tools aid in forensic reconstructions, investigative efforts, and the development of countermeasures against explosive threats.
Nanotechnology-Based Sensors: Nanotechnology-based sensors show promise for the development of highly sensitive and selective detection platforms for explosive analysis. Nanomaterials, such as carbon nanotubes, quantum dots, and metal-organic frameworks, can be functionalized to selectively bind to explosive molecules, enabling their detection at trace levels.
When we talk about India, It has made significant strides in the field of explosives analysis, leveraging technological advancements and research initiatives to enhance capabilities in forensic science, law enforcement, and national security. Several innovative approaches and developments have emerged in recent years, contributing to the detection, identification, and analysis of explosive materials. Here are some notable innovations in India in the field of explosives analysis:
Development of Portable Detection Technologies:
Indian researchers and organizations have been actively involved in the development of portable and handheld detection technologies for explosives. Efforts have focused on the creation of compact and lightweight devices that can rapidly detect trace amounts of explosive residues in various environments, including airports, railway stations, and public spaces. These portable detection technologies utilize techniques such as ion mobility spectrometry (IMS), gas chromatography-mass spectrometry (GC-MS), and fluorescence spectroscopy for sensitive and selective detection of explosive compounds.
Advancements in Spectroscopic Techniques:
Indian scientists have made advancements in spectroscopic techniques for the analysis of explosive materials. Research efforts have focused on the development of techniques such as Raman spectroscopy, Fourier-transform infrared (FTIR) spectroscopy, and laser-induced breakdown spectroscopy (LIBS) for the rapid and non-destructive identification of explosive residues.
These spectroscopic techniques offer high specificity and sensitivity, allowing for accurate analysis of complex mixtures and concealed explosives.
Use of Nanotechnology:
Nanotechnology has been increasingly employed in explosives analysis in India, offering novel approaches for detection and mitigation. Indian researchers have explored the use of nanostructured materials, such as carbon nanotubes, metal nanoparticles, and quantum dots, for the development of highly sensitive sensors and detectors. Nanomaterial-based sensors exhibit enhanced surface-to-volume ratios and unique optical, electrical, and catalytic properties, enabling the detection of trace amounts of explosive compounds with high selectivity.
Computational Modeling and Simulation:
Indian scientists have utilized computational modeling and simulation techniques to study the behavior of explosive materials and predict their properties. Computational approaches, including molecular dynamics simulations, density functional theory (DFT), and finite element analysis (FEA), have been employed to understand the mechanisms of explosive reactions, energetics, and detonation dynamics. These simulations provide valuable insights into the structure-property relationships of explosives, aiding in the design and optimization of new materials with tailored properties.
Collaboration and Interdisciplinary Research:
Collaborative efforts between academic institutions, research laboratories, and government agencies in India have facilitated interdisciplinary research in explosives analysis.
Joint research projects and partnerships have fostered knowledge exchange, technology transfer, and capacity building in the field of forensic science and explosives investigation.
Collaborative initiatives have led to the development of innovative analytical methods, instrumentation, and protocols for the analysis of explosive materials, addressing the evolving challenges posed by terrorist threats and criminal activities.
Conclusion:
The preliminary and confirmatory examination of explosives is a meticulous and systematic process that relies on a combination of scientific expertise, analytical techniques, and forensic methodologies. By following established protocols and utilizing state-of-the-art instrumentation, forensic scientists can accurately identify and characterize explosive materials, contributing to the investigation and prosecution of explosive-related crimes. This rigorous examination serves to uphold the principles of justice, protect public safety, and mitigate the risks associated with explosive incidents. The analysis of explosives is a complex and multifaceted endeavor that requires a combination of scientific expertise, advanced instrumentation, and innovative methodologies. From the identification of explosive residues at the scene of an explosion to the characterization of complex explosive mixtures in the laboratory, forensic scientists play a crucial role in unraveling the mysteries surrounding explosive incidents and ensuring public safety. As technology continues to advance and threats evolve, the field of explosive analysis remains at the forefront of scientific inquiry, driven by a relentless pursuit of truth, justice, and security.
Related Posts
Successfully Catering Events: From Planning to Execution
The world of event catering is a dynamic one, filled with delicious possibilities and the satisfying joy of creating memorable experiences. Whether you’re a seasoned
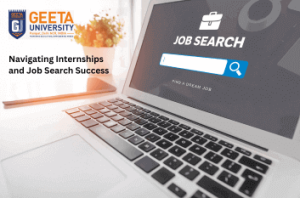
Navigating Internships and Job Search Success – Geeta University
Navigating Internships and Job Search Success – Geeta University Transitioning from the classroom to the professional world can be an exciting but challenging journey for
Navigating the Dynamics of Hydrology Engineering: Balancing Nature and Development
Navigating the Dynamics of Hydrology Engineering: Balancing Nature and Development Introduction: Hydrology engineering is a multifaceted discipline that plays a critical role in managing water