Introduction
- May 17, 2024
- Geeta University
In the intricate dance of agriculture, genetics and plant breeding hold the spotlight. They are the orchestrators of yield, resilience, and quality in crops, weaving the fabric of sustainable agriculture. As we delve into the realm of genetics and plant breeding, we uncover not only the fundamental principles guiding crop improvement but also the innovative techniques revolutionizing the field. Join me on this journey through the genetic landscape, where science meets the soil to cultivate a greener future.
The world’s population is burgeoning, and with it, the demand for food is reaching unprecedented levels. To meet this demand sustainably, agriculture must undergo a transformation. Genetics and plant breeding emerge as indispensable tools in this endeavor, offering the promise of crops that are not only high-yielding but also resilient to the challenges posed by climate change, pests, and diseases. At its core, genetics is the study of heredity and variation in organisms. Genes, the units of heredity, carry the blueprint for traits ranging from size and shape to disease resistance and nutritional content. In the context of plant breeding, genetics provides the foundation upon which breeders can manipulate traits to improve crop performance.
Genetic variation, the raw material of evolution, serves as the cornerstone of plant breeding. Within and among plant populations, natural variations offer a treasure trove of traits waiting to be explored. By tapping into this diversity, breeders can introduce novel traits into crop genomes, enriching their genetic pool and enhancing the resilience of agricultural systems. The field of plant breeding encompasses a spectrum of methodologies, from age-old techniques to cutting-edge technologies. Traditional breeding relies on the principles of selection and hybridization, where plants with desirable traits are chosen as parents to propagate those traits in offspring. While effective, this method often entails long breeding cycles and relies heavily on trial and error.
In recent decades, however, advances in molecular biology and genomics have revolutionized plant breeding. Techniques such as marker-assisted selection (MAS), genetic engineering, and genome editing offer precise tools to manipulate genes and accelerate breeding cycles. These methods enable breeders to bypass the limitations of traditional breeding, targeting specific genes for enhancement or modification with unprecedented precision. Moreover, the advent of genomics has ushered in a new era of discovery in plant breeding. By sequencing and analyzing entire genomes, researchers gain comprehensive insights into the genetic makeup of crops, unraveling the complex networks governing traits of interest. In order to find candidate genes linked to desired features, scientists must sort through enormous volumes of genomic data, which is made possible by bioinformatics tools.
The applications of genetics in plant breeding are diverse and far-reaching, addressing a myriad of challenges facing agriculture. From enhancing yield and nutritional quality to bolstering resilience against biotic and abiotic stresses, genetic interventions offer solutions to feed a growing population in a changing climate. Despite the promise of genetics in plant breeding, challenges and ethical considerations loom large. Concerns surrounding genetic diversity, intellectual property rights, and the potential environmental and health impacts of genetically modified organisms (GMOs) underscore the need for thoughtful regulation and oversight.
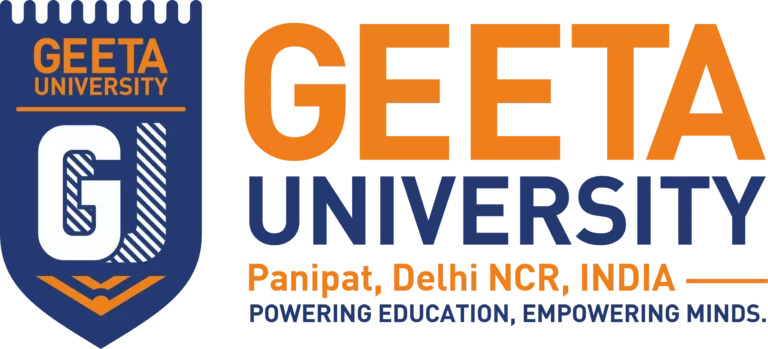
Admission Open 2024-2025
For Your bright Future
Understanding Genetics in Plant Breeding
At the heart of plant breeding lies genetics, the study of heredity and variation in organisms. It serves as the guiding light for breeders, offering insights into the inheritance patterns of traits and providing the framework for crop improvement. To comprehend the intricate interplay between genetics and plant breeding, let us explore the fundamental principles that underpin this dynamic relationship.
Genes: The Blueprint of Life
Genes are the fundamental units of heredity, encoding the instructions for the development and functioning of organisms. In plants, genes determine traits such as growth habit, flowering time, disease resistance, and nutrient content. Each gene occupies a specific location on a chromosome, and different versions of the same gene, called alleles, can result in variations in trait expression.
Inheritance Patterns: Mendelian Genetics
The basis for comprehending how traits are passed down from parents to children is provided by the concepts of Mendelian genetics, which were developed by Gregor Mendel in the 1800s. Mendel’s laws state that distinct units of inheritance, or genes, independently segregate and assort during gamete formation to determine a trait’s characteristics. Plant breeding techniques are based on the predictable patterns of inheritance that are produced by this, such as dominant-recessive relationships and segregation ratios.
Genetic Variation: Fuel for Innovation
Genetic variation is the lifeblood of evolution and adaptation. Within plant populations, genetic diversity arises from mutations, recombination, and gene flow, leading to differences in traits among individuals. This variation serves as the raw material for plant breeding, allowing breeders to select and cross plants with desirable traits to create new varieties with improved characteristics. By harnessing genetic variation, breeders can enhance crop performance, resilience, and adaptability to changing environmental conditions.
Quantitative Genetics: Beyond Mendel
While Mendelian genetics explains the inheritance of discrete traits controlled by a single gene, many important traits in plants are complex and influenced by multiple genes and environmental factors. Quantitative genetics provides the framework for understanding the inheritance of such polygenic traits, where variation is controlled by the cumulative effects of multiple genes. Through quantitative trait analysis, breeders can dissect the genetic basis of complex traits and develop breeding strategies to improve them.
Genetic Mapping: Unraveling the Genome
Advances in molecular biology and genomics have revolutionized our ability to dissect and manipulate the plant genome. Genetic mapping techniques, such as linkage mapping and association mapping, allow researchers to identify the genomic regions associated with specific traits of interest. By pinpointing the genes underlying these traits, breeders can accelerate the breeding process through marker-assisted selection (MAS), where molecular markers linked to desired traits are used to select superior plants with greater efficiency.
Genomic Selection: Predicting Performance
Genomic selection is a cutting-edge breeding strategy that harnesses genomic information to predict the performance of individuals based on their genetic makeup. By training predictive models using large-scale genomic and phenotypic data, breeders can estimate the breeding value of plants and identify elite individuals for further breeding cycles. This data-driven approach enables more accurate selection decisions, leading to faster genetic gains and more efficient crop improvement.
Genome Editing: Rewriting the Code
Recent advances in genome editing technologies, such as CRISPR-Cas9, have opened up new possibilities for precise manipulation of the plant genome. Unlike traditional genetic engineering, which involves the insertion of foreign DNA, genome editing allows for targeted modifications of specific genes with unparalleled precision. This revolutionary technology holds immense promise for accelerating crop improvement by introducing precise changes to enhance desirable traits or confer resistance to pests and diseases.
Genetic Variation: The Key to Diversity
Genetic variation is the cornerstone of evolution and adaptation. In plant breeding, it serves as a treasure trove of traits waiting to be explored. Natural variations within and among plant populations offer a reservoir of genetic diversity, providing the raw material for breeding programs. By tapping into this diversity through techniques like germplasm collection and molecular markers, breeders can introduce novel traits into crop genomes, enriching their genetic pool.
Origins of Genetic Variation:
Genetic variation arises through a multitude of mechanisms, each contributing to the mosaic of diversity within and among plant populations. One of the primary sources of variation is mutation, the spontaneous changes in DNA sequences that give rise to new alleles and traits. Mutations can occur due to environmental factors such as radiation or chemical exposure, as well as errors during DNA replication. Genetic recombination, which takes place during meiosis, the process of gamete formation, is another significant mechanism. Homologous chromosomes exchange genetic material through crossing over and independent assortment, resulting in novel allele combinations in offspring. With each generation, this process generates diversity by rearranging the genetic deck. By adding new alleles to gene pools, gene flow—the transfer of genes between populations—also increases genetic variation. Gene flow makes it easier for genetic material to be exchanged and encourages genetic mixing between populations, whether through human-mediated transport, pollen dispersal, or seed dissemination. Selective pressures exerted by the environment further shape genetic variation, favoring individuals with traits that confer a fitness advantage in specific ecological niches. Natural selection acts as a filter, allowing individuals with adaptive traits to survive and reproduce, while less fit individuals are gradually eliminated from the population.
Consequences of Genetic Variation:
Genetic variation is the currency of evolution, providing the raw material upon which natural selection acts. It fuels the process of adaptation, enabling populations to respond to changing environmental conditions and ecological challenges. In heterogeneous environments, diverse populations are better equipped to exploit available resources and withstand biotic and abiotic stresses. In agricultural systems, genetic variation is equally vital, serving as the foundation for crop improvement and breeding efforts. By tapping into the genetic diversity present within and among crop species, breeders can introduce novel traits into cultivated varieties, enhancing their resilience, productivity, and quality. Moreover, genetic variation underpins the concept of gene banks and germplasm collections, which serve as repositories of genetic diversity for future generations. These repositories safeguard endangered species, landraces, and wild relatives of crops, preserving valuable genetic resources that may hold the key to future agricultural challenges.
Harnessing Genetic Variation:
Utilizing genetic variation to create better crop varieties that satisfy the demands of farmers, consumers, and the environment is a critical task for plant breeders. Through selective breeding, breeders identify and propagate plants with desirable traits, such as high yield, disease resistance, or nutritional quality. This process involves crossing diverse parental lines to create hybrid offspring with complementary traits, followed by rigorous selection and evaluation of progeny. Modern breeding techniques, such as molecular markers and genomic selection, offer powerful tools for harnessing genetic variation with precision and efficiency. Molecular markers, such as single nucleotide polymorphisms (SNPs) or simple sequence repeats (SSRs), enable breeders to track desired traits at the DNA level, speeding up the selection process and reducing the need for labor-intensive phenotypic evaluations. Genomic selection takes this a step further by leveraging genomic information to predict the breeding value of individuals based on their genetic makeup. Breeders can select elite individuals for additional breeding cycles and estimate the performance of potential parents by training statistical models with large-scale genomic and phenotypic data. This data-driven strategy speeds up the rate of genetic gain in breeding programs and allows for more precise selection decisions.
Breeding Methods: From Conventional to Cutting-edge
Plant breeding is an ever-evolving field that uses a variety of approaches, from traditional methods to state-of-the-art technologies. Breeders are able to address the issues of agriculture and food security by creating new crop varieties with improved traits thanks to these varied approaches. Let’s explore the continuum of breeding methods, from conventional to cutting-edge:
Conventional Breeding:
Conventional breeding, also known as classical breeding, forms the backbone of plant breeding and has been practiced for thousands of years. It relies on the principles of selection and hybridization to improve crop varieties through controlled mating and selection of superior individuals. Breeders identify plants with desirable traits, such as high yield, disease resistance, or quality characteristics, and cross them to produce offspring with combined traits. Through repeated cycles of selection and hybridization, breeders create new varieties tailored to specific environmental conditions and market preferences.
Mass Selection:
In order to improve populations for traits that are challenging to measure individually, mass selection is a straightforward and intuitive breeding technique. Breeders choose and propagate a portion of plants from a population using mass selection, focusing less on individual traits and more on overall performance or phenotype. This method works especially well for enhancing low-heritability traits, like complex agronomic traits or environmental adaptability.
Pedigree Breeding:
Pedigree breeding, also known as pedigree selection or family-based selection, involves tracking the ancestry and performance of individual plants or families over multiple generations. Breeders maintain detailed records of parentage and performance data, allowing them to make informed decisions about which individuals to select as parents for the next generation. Pedigree breeding is commonly used in crops with long breeding cycles, such as tree fruits and perennial crops, where accurate evaluation of genetic potential is essential.
Hybridization:
Hybridization is a breeding technique that involves crossing two genetically distinct parental lines to produce offspring with hybrid vigor or heterosis. Hybrid varieties often exhibit superior performance compared to their parents, including increased yield, uniformity, and vigor. Hybridization is widely used in crops such as maize, rice, and vegetables, where hybrid vigor can lead to significant improvements in productivity and performance.
Marker-assisted Selection (MAS):
Marker-assisted selection (MAS) is a molecular breeding technique that leverages DNA markers to accelerate the breeding process and improve selection efficiency. Breeders can identify and choose plants with desired traits using MAS based on the presence or lack of molecular markers associated with those traits. Breeders can identify elite individuals more quickly and accurately than with traditional phenotypic selection methods by screening large populations of plants using molecular markers.
Genomic Selection:
Genomic selection is a state-of-the-art breeding strategy that utilizes genome-wide DNA markers and statistical models to predict the breeding value of individuals based on their genetic makeup. By analyzing large-scale genomic and phenotypic data from breeding populations, genomic selection enables breeders to estimate the genetic merit of individuals for traits of interest, such as yield, disease resistance, or quality characteristics. This data-driven approach facilitates more accurate selection decisions and accelerates the rate of genetic gain in breeding programs.
Genome Editing:
Genome editing technologies, such as CRISPR-Cas9, have revolutionized plant breeding by offering precise tools for targeted modification of the plant genome. Unlike traditional genetic engineering, which involves the insertion of foreign DNA, genome editing allows breeders to make precise changes to specific genes or DNA sequences without introducing foreign DNA. This enables the precise manipulation of traits to enhance desirable characteristics or confer resistance to pests, diseases, or environmental stresses.
Genomics and Bioinformatics: Decoding the Plant Genome
In the era of modern plant breeding, genomics and bioinformatics emerge as indispensable tools for unraveling the mysteries of the plant genome. These disciplines empower researchers to decipher the complex genetic codes that govern plant traits, unlocking a wealth of information that drives innovation in crop improvement. Let’s delve into the fascinating world of genomics and bioinformatics and their transformative impact on decoding the plant genome:
Genome Sequencing:
Genome sequencing is the cornerstone of genomics, providing a blueprint of the entire DNA sequence of an organism. Advances in sequencing technologies, such as next-generation sequencing (NGS) and third-generation sequencing (long-read sequencing), have made it feasible to sequence plant genomes with unprecedented speed, accuracy, and cost-effectiveness. By decoding the sequence of nucleotides comprising the plant genome, researchers gain insights into its structure, organization, and function, laying the foundation for further genomic analysis and exploration.
Genome Assembly:
Genome assembly is the process of reconstructing the complete genome sequence from the short DNA fragments generated by sequencing technologies. This computational challenge involves piecing together millions to billions of DNA reads into contiguous sequences called contigs, which represent the individual chromosomes or genomic regions of the organism. Bioinformatics tools and algorithms play a crucial role in genome assembly, helping researchers navigate the complexities of genomic data and assemble high-quality reference genomes for diverse plant species.
Annotation of Genomic Features:
Genomic annotation is the process of identifying and characterizing the functional elements encoded within the genome, including protein-coding genes, non-coding RNAs, regulatory elements, and repetitive sequences. Bioinformatics tools, such as gene prediction algorithms and sequence homology searches, enable researchers to annotate genomic features and assign putative functions to genes based on similarities to known sequences in databases. This annotation provides valuable insights into the genetic architecture of plants and facilitates the discovery of genes underlying important traits for crop improvement.
Comparative Genomics:
Comparative genomics is a powerful approach that involves comparing the genome sequences of different plant species to identify similarities, differences, and evolutionary relationships. By analyzing conserved genomic regions and gene families across diverse taxa, researchers can infer the evolutionary history of plants, trace the origins of key traits, and uncover genomic innovations that drive adaptation and speciation. Comparative genomics also enables the transfer of knowledge and genetic resources between related species, facilitating crop improvement through the identification of orthologous genes and genomic regions associated with desirable traits.
Functional Genomics:
Functional genomics aims to elucidate the functions of genes and regulatory elements in the genome, as well as their roles in biological processes and phenotypic traits. This multidisciplinary field integrates genomic, transcriptomic, proteomic, and metabolomic approaches to study gene expression, protein function, and metabolic pathways on a genome-wide scale. By employing high-throughput experimental techniques, such as RNA sequencing (RNA-seq) and chromatin immunoprecipitation sequencing (ChIP-seq), researchers can unravel the molecular mechanisms underlying complex traits and identify candidate genes for crop improvement.
Genome-wide Association Studies (GWAS):
Genome-wide association studies (GWAS) are powerful tools for identifying genetic variants associated with complex traits in natural or breeding populations. By genotyping a large number of individuals and correlating their genetic variation with phenotypic variation, researchers can pinpoint genomic regions harboring alleles that contribute to trait variation. Bioinformatics methods, such as statistical association tests and genotype-phenotype association analyses, enable the identification of candidate genes and regulatory elements underlying target traits, providing valuable insights for marker-assisted selection and genomic prediction in breeding programs.
Evolutionary Genomics and Phylogenomics:
Evolutionary genomics and phylogenomics explore the evolutionary history and relationships among plant species by analyzing their genomic sequences. These approaches utilize phylogenetic methods, molecular dating techniques, and comparative genomic analyses to reconstruct the evolutionary tree of life and infer ancestral relationships between taxa. By elucidating the patterns of genome evolution, gene duplication, and speciation events, evolutionary genomics provides a framework for understanding the genetic basis of biodiversity and adaptation in plants.
Applications of Genetics in Plant Breeding
Plant breeding relies heavily on genetics, which has many uses for enhancing crop quality, resilience, and productivity. Genetic interventions provide novel answers to the problems facing agriculture, from improving yield and nutritional content to boosting resistance against pests and diseases. Let’s explore some of the key applications of genetics in plant breeding:
1. Yield Improvement:
One of the primary objectives of plant breeding is to enhance crop yield to meet the growing demands of a global population. Genetics enables breeders to identify and incorporate traits associated with increased yield potential, such as larger grain size, improved biomass accumulation, and enhanced photosynthetic efficiency. By selecting and crossing high-yielding parental lines, breeders can develop varieties with superior productivity and performance under diverse environmental conditions.
2. Disease Resistance:
Plant diseases pose significant threats to agricultural productivity, causing yield losses and economic damage worldwide. Genetics offers strategies for developing crops with enhanced resistance to pathogens, including bacteria, fungi, viruses, and nematodes. Through the identification of resistance genes and the use of molecular markers, breeders can introgress genetic resistance into susceptible varieties, creating cultivars with durable and broad-spectrum resistance to diseases.
3. Pest Resistance:
Insect pests represent another major challenge for agriculture, requiring innovative solutions to mitigate their impact on crop production. Genetics provides avenues for developing crops with built-in resistance to insect pests through the expression of insecticidal proteins, such as Bacillus thuringiensis (Bt) toxins. Genetic engineering techniques allow breeders to introduce Bt genes into crop genomes, conferring protection against target pests while minimizing the need for chemical pesticides.
4. Abiotic Stress Tolerance:
Abiotic stresses, such as drought, salinity, heat, and cold, pose significant threats to crop production, particularly in the face of climate change. Genetics offers strategies for developing crops with enhanced tolerance to these environmental stresses, enabling them to thrive under adverse growing conditions. By identifying genes and genomic regions associated with stress tolerance traits, breeders can select and breed for varieties that exhibit improved resilience and performance in challenging environments.
5. Nutritional Enhancement:
Genetics plays a crucial role in the nutritional enhancement of crops, addressing deficiencies in essential vitamins, minerals, and micronutrients in human diets. Through biofortification, breeders can increase the levels of key nutrients, such as vitamin A, iron, zinc, and folate, in staple food crops like rice, wheat, maize, and beans. Genetic engineering techniques, such as transgenic approaches and genome editing, enable the precise manipulation of metabolic pathways to enhance nutrient accumulation in edible plant tissues.
6. Quality Improvement:
In addition to yield and nutritional traits, genetics also influences the quality attributes of crops, including taste, texture, color, and shelf life. Breeders utilize genetic variation to select for desirable quality traits, such as flavor, aroma, cooking characteristics, and post-harvest storage qualities. Through traditional breeding methods and molecular breeding approaches, breeders can develop varieties that meet consumer preferences and market demands, ensuring the production of high-quality agricultural products.
7. Environmental Sustainability:
Genetics contributes to environmental sustainability in agriculture by reducing the reliance on chemical inputs, minimizing environmental impacts, and promoting sustainable farming practices. Disease-resistant and pest-resistant varieties require fewer chemical pesticides, leading to reduced pesticide usage and environmental contamination. Similarly, stress-tolerant varieties can thrive in marginal lands with limited water and nutrient resources, promoting the efficient use of natural resources and reducing agricultural inputs.
Challenges and Ethical Considerations
Despite the promise of genetics in plant breeding, challenges and ethical considerations loom large. Concerns surrounding genetic diversity, intellectual property rights, and the potential environmental and health impacts of genetically modified organisms (GMOs) underscore the need for thoughtful regulation and oversight.
Furthermore, the unequal distribution of genetic resources and the concentration of breeding efforts in a few major crops raise questions of equity and access to innovation. Addressing these challenges requires a collaborative and inclusive approach, balancing scientific advancement with ethical considerations and societal needs.
Conclusion
In the intricate tapestry of agriculture, genetics and plant breeding emerge as powerful tools for shaping the future of food production. From unraveling the mysteries of inheritance to harnessing the potential of genomics, breeders continue to push the boundaries of innovation in their quest for sustainable solutions. As we navigate the complex landscape of genetics and plant breeding, let us tread carefully, guided by principles of equity, sustainability, and ethical stewardship. Together, we can cultivate a greener, more resilient future for generations to come.
Tags
Related Posts
Unveiling the Power of Genetics in Plant Breeding: A Pathway to Sustainable Agriculture
Introduction In the intricate dance of agriculture, genetics and plant breeding hold the spotlight. They are the orchestrators of yield, resilience, and quality in crops, weaving the fabric of sustainable agriculture. As we delve into the realm of genetics and