- May 22, 2024
- Geeta University
Micromeritics is a branch of science and technology focused on the study of small particles, typically ranging from nanometers to micrometers in size, and their behaviour in various systems and environments. It encompasses a wide range of techniques and methodologies aimed at characterizing the physical and chemical properties of these particles. These properties include particle size distribution, surface area, pore size distribution, pore volume, density, and other related parameters.
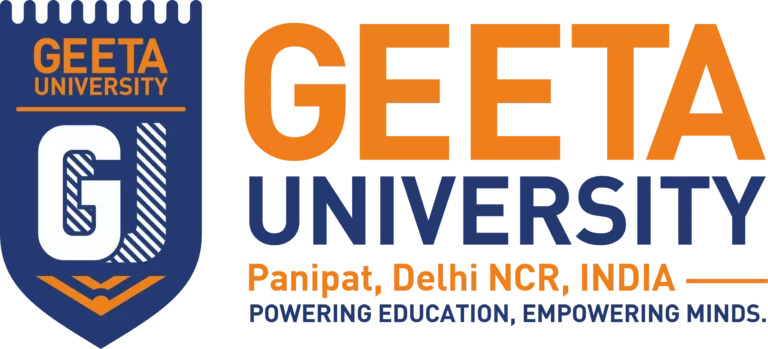
Admission Open 2024-2025
For Your bright Future
The study of micromeritics is essential across numerous industries and research fields due to the pervasive presence and importance of small particles. Some of the key areas where micromeritics plays a crucial role include:
Pharmaceuticals: In pharmaceutical formulation, understanding the particle size distribution and surface area of active pharmaceutical ingredients (APIs) and excipients is critical for optimizing drug delivery systems, such as tablets, capsules, and suspensions. Micromeritics techniques help ensure the efficacy, stability, and bioavailability of pharmaceutical products.
Chemicals: Many chemical processes involve particulate systems, where the properties of catalysts, reactants, and products depend on particle characteristics. Micromeritics analysis aids in catalyst development, powder synthesis, and process optimization in industries such as catalysis, pigments, and additives.
Materials Science: The properties of materials such as powders, nanoparticles, and porous materials are deeply influenced by their microstructure. Micromeritics techniques provide valuable insights into the structure-property relationships of materials, guiding the design and development of advanced materials for applications in electronics, energy storage, coatings, and composites.
Environmental Science: Understanding the characteristics of particulate matter in environmental samples is crucial for assessing air and water quality, pollutant transport, and environmental remediation efforts. Micromeritics methods help quantify particle size distribution, surface area, and pore structure in environmental samples, aiding in pollution monitoring and management.
Techniques of Micromeritics
Particle Size Analysis: Various methods, such as laser diffraction, dynamic light scattering, and microscopy, are employed to determine the size distribution of particles in a sample.
Surface Area Analysis: Techniques like BET (Brunauer-Emmett-Teller) method and gas adsorption are used to measure the surface area of porous materials by adsorbing gas molecules onto the surface.
Pore Size Distribution and Volume Analysis: Methods such as mercury intrusion porosimetry and gas adsorption help characterize the pore structure of materials by measuring the volume of pores and their size distribution.
Density Measurement: Techniques like gas pycnometry and helium pycnometry are used to determine the true density, apparent density, and bulk density of powders and porous materials.
Overall, micromeritics plays a crucial role in understanding and manipulating the behavior of small particles, enabling advancements in various fields and industries where particulate systems are involved.
Analysis of micromeritics
Sieve Analysis: Particles are separated by size using a series of sieves with progressively finer mesh sizes. The weight of particles retained on each sieve is measured to determine the particle size distribution.
Laser Diffraction: A laser beam is directed at particles in suspension, and the pattern of light scattered by the particles is analyzed to determine particle size distribution.
Dynamic Light Scattering (DLS): Measures the fluctuations in the intensity of light scattered by particles in Brownian motion to determine particle size distribution, especially effective for nanoparticles.
Microscopy Methods: Direct visualization of particles using optical microscopy, scanning electron microscopy (SEM), or transmission electron microscopy (TEM) to determine particle size and shape.
Surface Area Analysis:
Brunauer-Emmett-Teller (BET) Method: Gas adsorption (commonly nitrogen) is used to measure the surface area of porous materials based on the amount of gas adsorbed onto the surface.
Langmuir Method: Similar to the BET method, measures gas adsorption to determine surface area but under assumptions different from the BET method.
Single-Point and Multi-Point BET: Variants of the BET method used depending on the specific characteristics of the material being analyzed.
Pore Size Distribution and Pore Volume Analysis:
Mercury Intrusion Porosimetry (MIP): Mercury is forced into the pores of a material at various pressures, and the volume of mercury intruded is measured to determine pore size distribution and total pore volume.
Gas Adsorption Methods: Gas adsorption techniques such as BET can also provide information about pore size distribution, especially for mesoporous and microporous materials.
Capillary Flow Porometry: Measures the flow of a wetting liquid through a porous material to determine pore size distribution.
Density Measurement:
Gas Pycnometry: Measures the volume of a sample by comparing the volume occupied by a gas at known pressure and temperature with that occupied by the sample.
Helium Pycnometry: Similar to gas pycnometry but uses helium as the displacing gas, especially useful for materials with low density or porosity.
Flow property of powders:-
The flow properties of powders refer to how well a powder material can flow or move under various conditions. These properties are crucial in industries such as pharmaceuticals, food processing, cosmetics, and chemicals, where powders are commonly handled, processed, and manufactured. Poor flow properties can lead to problems such as uneven mixing, inconsistent dosing, and difficulties in packaging and handling. Understanding and optimizing flow properties are essential for ensuring efficient processing and high-quality end products. Some key aspects of flow properties include:
Flowability: Flowability refers to the ease with which a powder can flow under the influence of gravity. Factors influencing flowability include particle size, shape, density, surface properties, moisture content, and interparticle forces. Powders with good flowability exhibit smooth, uniform flow without clumping, segregation, or blockages.
Angle of Repose: The angle of repose is the maximum angle formed between the surface of a pile of powder and the horizontal plane. It provides a measure of the powder’s flowability and cohesion. Powders with low angles of repose tend to flow more freely, while those with high angles of repose may exhibit poor flow properties.
Cohesion: Cohesion refers to the tendency of powder particles to stick together due to interparticle forces such as van der Waals forces, electrostatic forces, and capillary forces. High cohesion can result in poor flowability, leading to issues such as arching, bridging, and rat-holing during handling and processing.
Segregation: Segregation occurs when particles of different sizes, shapes, or densities separate during handling or flow, leading to non-uniform distribution of components within a powder mixture. Factors such as particle size distribution, density differences, and flow conditions influence segregation behavior.
Bulk Density: Bulk density is the mass of a powder per unit volume, including the void spaces between particles. It affects the flowability, compressibility, and packaging density of powders. Low bulk density powders may exhibit poor flow properties, while high bulk density powders may be prone to caking and compaction.
Compressibility: Compressibility refers to the ability of a powder to change its volume under pressure. It influences the flow behavior during compaction, tableting, and packaging processes. Powders with low compressibility tend to flow more easily and uniformly, while highly compressible powders may exhibit erratic flow behavior.
Powder Flow Testing: Various methods and instruments are available for assessing the flow properties of powders, including flowability testers, shear testers, tapped density testers, and powder rheometers. These instruments measure parameters such as flow rate, flow energy, cohesion index, and flow function to characterize powder flow behavior quantitatively.
Optimizing the flow properties of powders often involves modifying particle size distribution, shape, surface properties, moisture content, and formulation additives. By understanding and controlling these factors, manufacturers can improve process efficiency, product quality, and consistency in powder handling and processing operations.
Future aspects of micromeritics
Nanomaterials Characterization: With the increasing importance of nanotechnology in various industries, there will be a growing demand for advanced techniques to characterize nanoparticles and nanostructured materials. Micromeritics methods will continue to evolve to address the unique challenges associated with characterizing nanoscale materials, such as precise control over particle size, shape, surface properties, and dispersibility.
In situ and Operando Analysis: There is a growing interest in studying materials under real-world operating conditions to understand dynamic changes in structure and properties. In situ and operando micromeritics techniques, coupled with advanced imaging and spectroscopic methods, will enable researchers to investigate phenomena such as catalyst dynamics, battery degradation, and drug release kinetics with unprecedented detail.
Multi-scale Modeling and Simulation: Integrating experimental data with computational modeling and simulation approaches will facilitate a deeper understanding of complex particle-particle and particle-environment interactions. Multi-scale modeling will enable researchers to predict macroscopic behavior based on microscopic parameters, aiding in the design and optimization of materials and processes.
Smart and Functional Materials Design: Micromeritics will play a vital role in the development of smart and functional materials with tailored properties for specific applications. By precisely controlling particle size, morphology, surface area, and porosity, researchers can design materials with enhanced performance in areas such as drug delivery, catalysis, energy storage, and sensing.
High-throughput Screening and Automation: The need for rapid and efficient characterization of large libraries of materials will drive the development of high-throughput micromeritics techniques and automated instrumentation. These advancements will enable researchers to accelerate materials discovery and optimization processes, leading to faster innovation cycles and reduced time-to-market for new products.
Environmental and Sustainability Applications: Micromeritics methods will continue to play a crucial role in addressing environmental and sustainability challenges by enabling the development of eco-friendly materials and processes. Characterizing the microstructure of materials used in pollution control, renewable energy, and waste management applications will aid in designing more efficient and sustainable solutions.
Integration with Industry 4.0 Technologies: Micromeritics instrumentation will increasingly be integrated with Industry 4.0 technologies such as internet of things (IoT), artificial intelligence (AI), and big data analytics. This integration will enable real-time monitoring, predictive maintenance, and data-driven decision-making in manufacturing processes, enhancing efficiency, quality control, and resource optimization.
Role of high throughput screening in micromeritics
Efficient Characterization of Particle Properties: HTS allows researchers to quickly analyze a wide range of micromeritics properties, including particle size distribution, surface area, pore size distribution, and density, across numerous samples. This enables comprehensive characterization of materials and provides valuable insights into their structure-property relationships.
Accelerated Materials Discovery and Development: By automating the process of particle property characterization, HTS accelerates materials discovery and development efforts. Researchers can screen large libraries of materials, formulations, or process conditions to identify promising candidates for further study, optimization, or commercialization.
Optimization of Manufacturing Processes: HTS facilitates the optimization of manufacturing processes by systematically evaluating the effects of process parameters on particle properties. Researchers can conduct high throughput experiments to identify optimal conditions for powder synthesis, formulation, granulation, compaction, and other manufacturing processes.
Quality Control and Assurance: HTS plays a crucial role in quality control and assurance by enabling rapid and comprehensive analysis of raw materials, intermediates, and finished products. Automated screening of particle properties ensures consistency, reproducibility, and compliance with specifications, leading to high-quality products and processes.
Exploration of Structure-Property Relationships: HTS enables researchers to explore complex structure-property relationships in materials by systematically varying material composition, particle morphology, processing conditions, and environmental factors. By correlating high throughput screening data with performance metrics, researchers can gain deeper insights into material behavior and functionality.
High-throughput Formulation Studies: In industries such as pharmaceuticals, cosmetics, and food, HTS facilitates high throughput formulation studies by rapidly evaluating the impact of formulation components, excipients, and processing parameters on particle properties. This enables the development of optimized formulations with desired performance attributes.
Screening of Nanomaterials and Advanced Materials: HTS is particularly valuable for screening nanomaterials and advanced materials with complex microstructures and properties. By efficiently characterizing nanomaterials across a large parameter space, researchers can identify novel materials with unique functionalities for various applications, including catalysis, drug delivery, energy storage, and sensors.
Integration of Artificial Intelligence and Machine Learning: HTS generates large volumes of data, and integrating artificial intelligence (AI) and machine learning (ML) algorithms can enhance data analysis, pattern recognition, and predictive modeling. AI-powered HTS platforms can autonomously design experiments, optimize screening protocols, and identify correlations between experimental variables and outcomes, leading to more efficient and effective screening processes.
Microfluidics and Lab-on-a-Chip Technologies: Microfluidics-based HTS platforms offer miniaturization, automation, and parallelization of experiments, allowing researchers to screen large libraries of compounds or samples with minimal reagents and resources. Lab-on-a-chip devices enable precise control over fluid flows, reaction conditions, and sample manipulation, enabling rapid and multiplexed screening of biological, chemical, and materials samples.
Multi-dimensional Screening Platforms: Future HTS platforms may incorporate multi-dimensional screening approaches that simultaneously evaluate multiple properties or parameters of interest. By integrating complementary screening assays, such as phenotypic, target-based, and biophysical assays, researchers can gain a more comprehensive understanding of complex biological systems, drug-target interactions, and material properties.
High-content Screening and Imaging Technologies: Advancements in imaging technologies, microscopy, and image analysis algorithms enable high-content screening (HCS) of cellular, subcellular, and tissue-level phenotypes. Future HTS platforms may integrate HCS capabilities to profile cellular responses, morphological changes, and biomolecular interactions in high throughput, facilitating drug discovery, functional genomics, and systems biology studies.
Automation and Robotics: Automation and robotics will continue to play a crucial role in HTS, enabling the rapid execution of experiments, sample handling, and data acquisition. Future HTS platforms may feature advanced robotic systems with enhanced dexterity, flexibility, and autonomy, allowing for seamless integration of multiple assays, liquid handling modules, and data management workflows.
High-throughput Screening in 3D and Organoid Models: Three-dimensional (3D) cell culture models and organoids represent more physiologically relevant systems for drug screening and toxicity testing compared to traditional monolayer cultures. Future HTS platforms may incorporate 3D culture technologies and organ-on-a-chip systems to screen compounds in complex tissue-like environments, offering insights into tissue physiology, disease mechanisms, and drug responses.
Open Access and Collaborative Platforms: Future HTS platforms may embrace open access and collaborative models, enabling researchers from diverse disciplines and organizations to access shared screening facilities, resources, and datasets. Collaborative HTS initiatives can foster innovation, knowledge exchange, and community-driven research efforts, leading to new discoveries and breakthroughs in biomedical, chemical, and materials sciences.
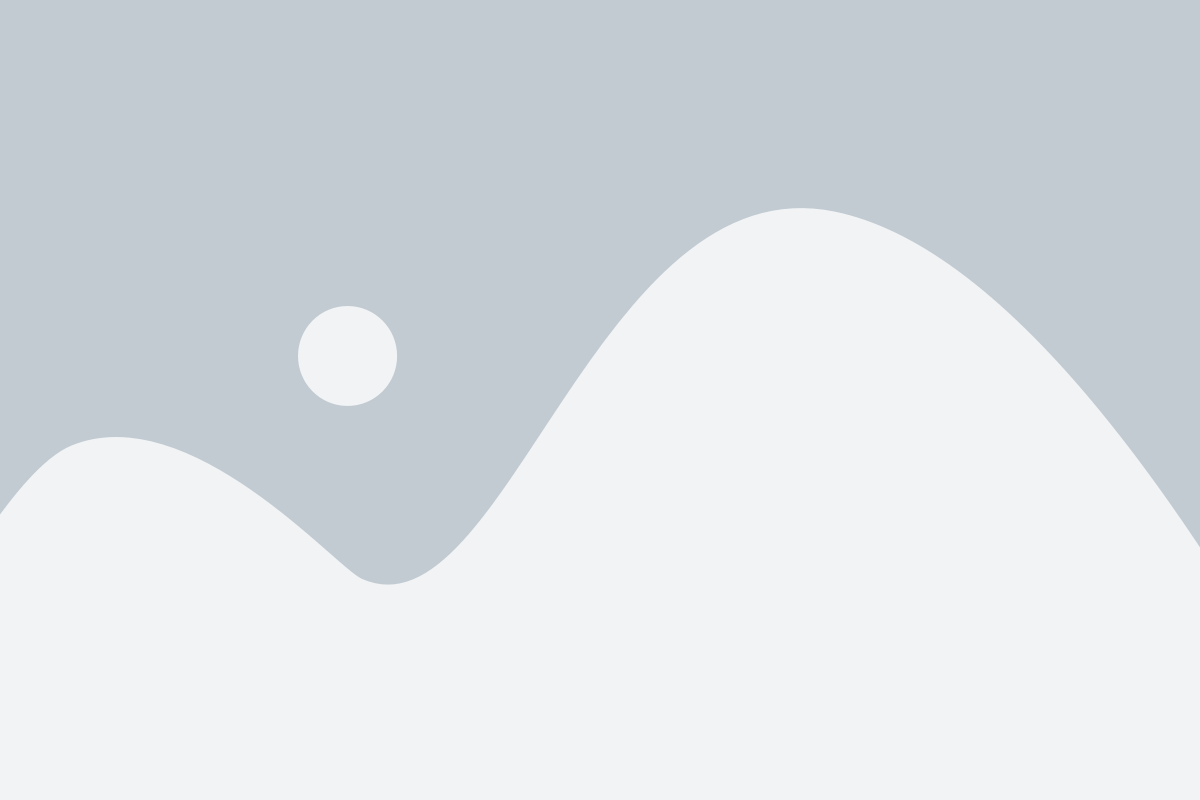
Ms. Twinkle
Assistant Professor, Geeta Institute of Pharmacy
Geeta University, Panipat
Tags
Related Posts
COCRYSTALS: APPLICATIONS IN PHARMACY
Introduction Many medicines with limited aqueous solubility have been found in the recent few years. Of the newly identified medications, between 60 and 70 percent of the compounds belong to BCS Class II (low solubility/high permeability) and IV (low solubility/low
Unveiling the Microcosm: Exploring Micromeritics for Precision Innovation
Micromeritics is a branch of science and technology focused on the study of small particles, typically ranging from nanometers to micrometers in size, and their behaviour in various systems and environments. It encompasses a wide range of techniques and methodologies
Nanocrystals gels: Polysacchrides and Inorganic
Introduction One kind of nanostructured material made up of scattered nanocrystals within a gel matrix is called a nanocrystal gel. By utilizing the special qualities of nanocrystals, these gels can produce materials with improved mechanical, optical, and electrical properties. Understanding