Advanced Composites and Hybrid Materials: Pioneering the Future of Engineering
- October 7, 2024
- Geeta University
Introduction
In the realm of modern engineering, the pursuit of materials that exhibit superior properties has led to the development of advanced composites and hybrid materials. These materials are not only shaping the future of industries such as aerospace, automotive, and construction but are also revolutionizing how we approach challenges related to sustainability, performance, and innovation. The journey from traditional materials to advanced composites and hybrids reflects the continuous quest for excellence in material science.
The Evolution of Materials in Engineering
Traditional Materials and Their Limitations
Historically, engineering relied heavily on metals, ceramics, and polymers, each of which offered distinct advantages and limitations. Metals, known for their strength and durability, often suffered from weight-related drawbacks. Ceramics provided excellent thermal and chemical resistance but were brittle. Polymers offered flexibility and corrosion resistance but lacked the mechanical strength required for many high-performance applications. These limitations paved the way for the development of composite materials that could combine the best properties of individual materials.
Understanding Advanced Composites
What are Advanced Composites?
Advanced composites are a class of composite materials that exhibit high performance in terms of strength, stiffness, weight, and durability. These materials are often used in applications where traditional composites would not meet the required performance standards. The matrix materials in advanced composites can include thermosetting polymers, thermoplastic polymers, ceramics, and even metals, while the reinforcement can consist of carbon fibers, aramid fibers, glass fibers, or other high-performance materials.
Types of Advanced Composites
1. Carbon Fiber Reinforced Polymers (CFRP): These are among the most widely used advanced composites. Carbon fibers provide high strength and stiffness, while the polymer matrix offers flexibility and ease of fabrication. CFRPs are used extensively in aerospace, automotive, and sports equipment due to their excellent strength-to-weight ratio.
2. Glass Fiber Reinforced Polymers (GFRP): GFRPs are less expensive than CFRPs and offer good mechanical properties and corrosion resistance. They are commonly used in construction, automotive components, and marine applications.
3. Aramid Fiber Reinforced Polymers (AFRP): Aramid fibers, such as Kevlar, provide exceptional impact resistance and energy absorption. AFRPs are used in applications requiring high toughness, such as ballistic protection and sporting goods.
4. Metal Matrix Composites (MMC): These composites consist of a metal matrix reinforced with fibers or particles. MMCs offer superior mechanical properties, including high-temperature resistance, making them suitable for aerospace and automotive engine components.
5. Ceramic Matrix Composites (CMC): CMCs are designed for extreme environments, such as high temperatures and corrosive conditions. They are used in aerospace applications, particularly in turbine engines, where traditional metals would fail.
The Role of Hybrid Materials
Defining Hybrid Materials
Hybrid materials represent a step further in the evolution of composites. They consist of two or more different types of materials combined at the molecular or macroscopic level to achieve unique properties that are not attainable with single-material composites. Hybrids can include combinations of organic and inorganic materials, polymers with metals or ceramics, and more. The aim is to tailor the properties of the material to meet specific application requirements.
Types of Hybrid Materials
1. Polymer-Metal Hybrids: These materials combine the flexibility and processability of polymers with the strength and thermal conductivity of metals. They are used in applications where lightweight and thermal management are critical, such as in electronics and automotive components.
2. Polymer-Ceramic Hybrids: These hybrids offer the flexibility of polymers with the thermal and chemical resistance of ceramics. They are used in applications such as protective coatings, electronics, and biomedical devices.
3. Metal-Ceramic Hybrids: These materials combine the high-temperature resistance and hardness of ceramics with the toughness and ductility of metals. They are used in applications such as cutting tools, aerospace components, and wear-resistant coatings.
4. Organic-Inorganic Hybrids: These materials combine organic polymers with inorganic materials at the molecular level. They offer a unique combination of properties, such as flexibility, optical clarity, and high thermal stability. Applications include optoelectronics, sensors, and coatings.
Applications of Advanced Composites and Hybrid Materials
Aerospace and Defense
The aerospace and defense industries are at the forefront of utilizing advanced composites and hybrid materials. The demand for lightweight, strong, and durable materials in these sectors is driven by the need to enhance fuel efficiency, reduce emissions, and improve performance. CFRPs are extensively used in aircraft structures, including fuselage, wings, and tail sections, where weight reduction without compromising strength is critical. In defense, advanced composites are used in armor systems, unmanned vehicles, and high-performance aircraft.
Automotive Industry
The automotive industry is increasingly adopting advanced composites and hybrid materials to address the challenges of weight reduction and fuel efficiency. CFRPs and GFRPs are used in the manufacture of car bodies, chassis, and interior components. The use of these materials helps reduce vehicle weight, which in turn improves fuel efficiency and reduces emissions. Hybrid materials, such as polymer-metal hybrids, are also used in automotive applications to provide a balance between strength and lightweight.
Construction and Infrastructure
In construction, advanced composites and hybrid materials are used to create structures that are strong, durable, and resistant to environmental factors such as corrosion and weathering. GFRPs are commonly used in bridges, beams, and reinforcing bars, where their lightweight and corrosion resistance provide significant advantages over traditional materials like steel. Hybrid materials are also being explored for use in smart structures that can monitor their own health and adapt to changing conditions.
Energy Sector
The energy sector, particularly wind energy, is a significant user of advanced composites. Wind turbine blades made from CFRPs and GFRPs offer the necessary strength and stiffness while keeping the overall weight of the turbine low. This is crucial for the efficiency and longevity of wind turbines. In addition, hybrid materials are being explored for use in energy storage systems, where they can offer improved performance and safety over traditional materials.
Medical and Biomedical Applications
Advanced composites and hybrid materials are also making significant contributions to the medical and biomedical fields. CFRPs and AFRPs are used in prosthetics and orthotics, where their lightweight and strength provide enhanced comfort and performance for patients. Hybrid materials are being developed for use in implants, drug delivery systems, and tissue engineering, where their ability to combine multiple functions, such as biocompatibility and mechanical strength, is essential.
Sports and Recreation
The sports and recreation industry has been quick to adopt advanced composites and hybrid materials to create high-performance equipment. CFRPs are used in the manufacture of bicycles, tennis rackets, golf clubs, and other sporting goods, where their strength, stiffness, and lightweight properties provide a competitive edge. Hybrid materials are also being used to create protective gear, such as helmets and pads, that offer superior impact resistance and comfort.
Challenges and Future Trends
Manufacturing and Cost Challenges
One of the main challenges associated with advanced composites and hybrid materials is the complexity of their manufacturing processes. The need for specialized equipment, stringent quality control, and skilled labor can make the production of these materials expensive. This, in turn, can limit their widespread adoption, particularly in cost-sensitive industries. However, ongoing research and development efforts are focused on reducing manufacturing costs through automation, process optimization, and the development of new materials that are easier to produce.
Recycling and Sustainability
As the use of advanced composites and hybrid materials grows, so does the need to address their end-of-life disposal and recycling. Traditional recycling methods are often unsuitable for these materials, particularly for composites that consist of different types of fibers and matrices. Developing sustainable recycling methods that can recover valuable materials without compromising their properties is an ongoing challenge. Additionally, there is a growing interest in developing bio-based composites and hybrids that can reduce the environmental impact of these materials.
Future Trends
The future of advanced composites and hybrid materials is set to be transformative, driven by technological advancements, evolving industrial needs, and global sustainability goals. Several key trends are expected to shape the development and application of these materials in the coming years. Below is a detailed exploration of these future trends.
1. Integration of Smart Features
a. Self-Healing Materials: One of the most exciting advancements in the realm of advanced composites is the development of self-healing materials. These materials can automatically repair damage such as cracks or abrasions without the need for external intervention. The application of self-healing materials could revolutionize industries like aerospace, where structural integrity is paramount. In addition to enhancing safety, self-healing materials can significantly reduce maintenance costs and downtime, as well as extend the lifespan of critical components.
b. Sensing and Actuation Capabilities: Smart composites are being developed with embedded sensors and actuators that allow them to monitor their own health and respond to environmental stimuli. For instance, piezoelectric materials embedded within a composite can generate an electric charge in response to mechanical stress, providing real-time data on the material’s condition. This capability is particularly valuable in applications such as aircraft wings, bridges, and other critical infrastructure, where early detection of damage can prevent catastrophic failures.
Actuators embedded within composites can enable these materials to change shape or stiffness in response to external stimuli, leading to applications in adaptive structures. For example, aerospace components made from smart composites could adjust their shape during flight to optimize aerodynamics and fuel efficiency.
c. Adaptive and Shape-Memory Materials: Shape-memory composites, which can return to a predefined shape after deformation, are another area of active research. These materials have potential applications in deployable structures, such as satellite antennas, that need to be compact during launch and then expand once in space. Additionally, adaptive materials that can alter their properties in response to environmental changes are being developed for use in smart textiles, biomedical devices, and responsive architecture.
2. Nanocomposites and Nanohybrids
a. Incorporation of Nanomaterials: Nanotechnology is playing a significant role in advancing the performance of composite materials. By incorporating nanomaterials such as carbon nanotubes (CNTs), graphene, and nanoclays into the matrix or reinforcement of composites, researchers are achieving remarkable enhancements in mechanical, electrical, and thermal properties.
b. Nanohybrids: Nanohybrids are materials that combine nanomaterials with other types of materials, such as polymers, metals, or ceramics, to achieve synergistic effects. For example, polymer nanohybrids that incorporate nanoparticles can exhibit enhanced mechanical strength, thermal stability, and UV resistance. Metal-ceramic nanohybrids, on the other hand, can offer superior wear resistance and high-temperature performance, making them suitable for cutting tools and aerospace components.
The potential applications of nanocomposites and nanohybrids are vast, ranging from lightweight, high-strength materials for aerospace to flexible, transparent conductors for next-generation electronics.
3. Customization and Personalization
a. Digital Manufacturing and 3D Printing: Advances in digital manufacturing techniques, particularly 3D printing (additive manufacturing), are enabling the creation of highly customized and personalized composite and hybrid materials. 3D printing allows for precise control over the material’s microstructure and composition, enabling the production of complex geometries and tailored properties that are difficult or impossible to achieve with traditional manufacturing methods.
For example, 3D printing can be used to create composite materials with graded properties, where the material’s composition changes gradually throughout its structure. This can result in components that combine flexibility and strength in a single part, such as a prosthetic limb that mimics the properties of human bone and muscle.
The ability to print customized composites also opens up new possibilities for personalized products, such as custom-fit medical implants, sports equipment tailored to an individual’s biomechanics, and bespoke automotive components designed for optimal performance.
b. Multi-Material Printing: Multi-material 3D printing is another emerging trend that allows for the simultaneous deposition of different materials within a single print. This capability enables the creation of hybrid materials with distinct regions of varying properties, such as stiffness, flexibility, or conductivity. Multi-material printing is particularly valuable for producing complex devices like wearable electronics, where different parts of the device need to perform different functions.
As 3D printing technology continues to evolve, we can expect to see greater integration of advanced composites and hybrid materials into the digital manufacturing ecosystem, leading to more efficient, sustainable, and innovative production processes.
4. Sustainability and Recycling
a. Bio-Based Composites and Hybrids: Sustainability is becoming an increasingly important consideration in the development of advanced materials. Researchers are exploring the use of bio-based materials, such as natural fibers (e.g., flax, hemp, jute) and bio-resins, to create eco-friendly composites. These bio-based composites offer a lower environmental impact compared to traditional composites made from petroleum-based materials, as they are derived from renewable resources and are often biodegradable or recyclable.
Bio-based composites are finding applications in automotive interiors, construction materials, and consumer goods. For example, natural fiber-reinforced composites are being used in car door panels, where their lightweight and sustainability offer advantages over conventional materials.
b. Recycling and Circular Economy: The recycling of advanced composites and hybrid materials remains a significant challenge due to the complexity of their composition. Traditional recycling methods, such as mechanical grinding, often degrade the material’s properties, limiting its reuse in high-performance applications. To address this issue, researchers are developing new recycling techniques, such as chemical recycling and thermochemical processes, that can break down composites into their constituent materials without compromising their quality.
In addition to recycling, the concept of a circular economy is gaining traction in the materials industry. This approach emphasizes the design of products and materials with their end-of-life in mind, enabling them to be reused, remanufactured, or recycled in a closed-loop system. For example, manufacturers are exploring the use of reversible adhesives and modular designs that allow composite structures to be easily disassembled and recycled.
The development of sustainable composites and hybrid materials is not only driven by environmental concerns but also by regulatory pressures and consumer demand for greener products. As such, we can expect to see a continued focus on sustainability in the materials industry, with innovations in bio-based materials, recycling technologies, and circular economy practices.
5. Advanced Manufacturing Techniques
a. Automated Fiber Placement (AFP) and Automated Tape Laying (ATL): Automated fiber placement (AFP) and automated tape laying (ATL) are advanced manufacturing techniques that are increasingly being used in the production of composite materials. These processes involve the automated placement of continuous fiber tapes or tows onto a mold or substrate, allowing for precise control over the fiber orientation and placement. This results in composite structures with optimized mechanical properties and minimal waste.
b. Out-of-Autoclave (OoA) Processing: Out-of-autoclave (OoA) processing is another trend in the manufacturing of composite materials. Traditional composite manufacturing often requires the use of an autoclave, a high-pressure oven that cures the composite material at elevated temperatures and pressures. However, autoclave processing can be expensive and energy-intensive.
c. Digital Twin and Simulation-Driven Design: The use of digital twins and simulation-driven design is becoming increasingly important in the development of advanced composites and hybrid materials. A digital twin is a virtual model of a physical object or system that can be used to simulate its behavior under different conditions. This allows engineers to optimize the design of composite materials and structures before they are physically manufactured.
Simulation-driven design enables the exploration of a wide range of material combinations and configurations, helping to identify the optimal design for a given application. This approach can also reduce the need for physical prototyping, saving time and resources in the development process.
As digital manufacturing and simulation technologies continue to advance, they will play a critical role in the development of next-generation composite and hybrid materials, enabling more efficient and innovative design processes.
Conclusion
Advanced composites and hybrid materials are at the forefront of material science innovation, offering a unique combination of properties that are driving progress across a wide range of industries. From aerospace and automotive to medical devices and sports equipment, these materials are enabling the development of products that are lighter, stronger, more durable, and more efficient than ever before. While challenges remain, particularly in the areas of manufacturing, cost, and sustainability, the future of advanced composites and hybrid materials is bright. Ongoing research and development efforts are likely to yield new materials and technologies that will further expand the possibilities of what can be achieved in engineering and beyond. As we continue to push the boundaries of material science, advanced composites and hybrid materials will undoubtedly play a crucial role in shaping the future of our world.
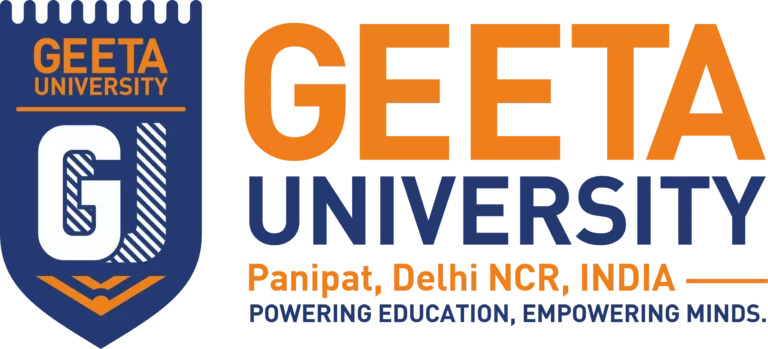
Admission Open 2024-2025
For Your bright Future
Tags
Related Posts
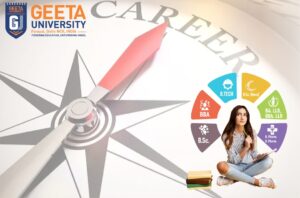
Why career guidance is important after 10th and 12th class – Geeta University
More than 95% students pass their class 10th and 12th Board examination, but how many of them make an effective choice post the completion of their respective educational milestones. It is rather significant to be mindful of the choices you
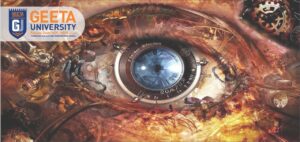
Best Courses After 12th Commerce, Future, Scope and Career Prospects – Geeta University
If you do Mathematics as an additional subject in classes 11 and 12, you are eligible for many courses as a second-degree qualified student. All of these subjects validate the need for Mathematics as a subject. In this competitive world,
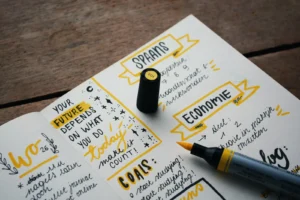
Diploma Courses in Engineering After Class 10th – Geeta University
Do you enjoy creating, designing, constructing, and maintaining tools and machines? If so, engineering is the ideal career for you. For those who desire to pursue technical education while also beginning their careers early, a diploma in engineering is a