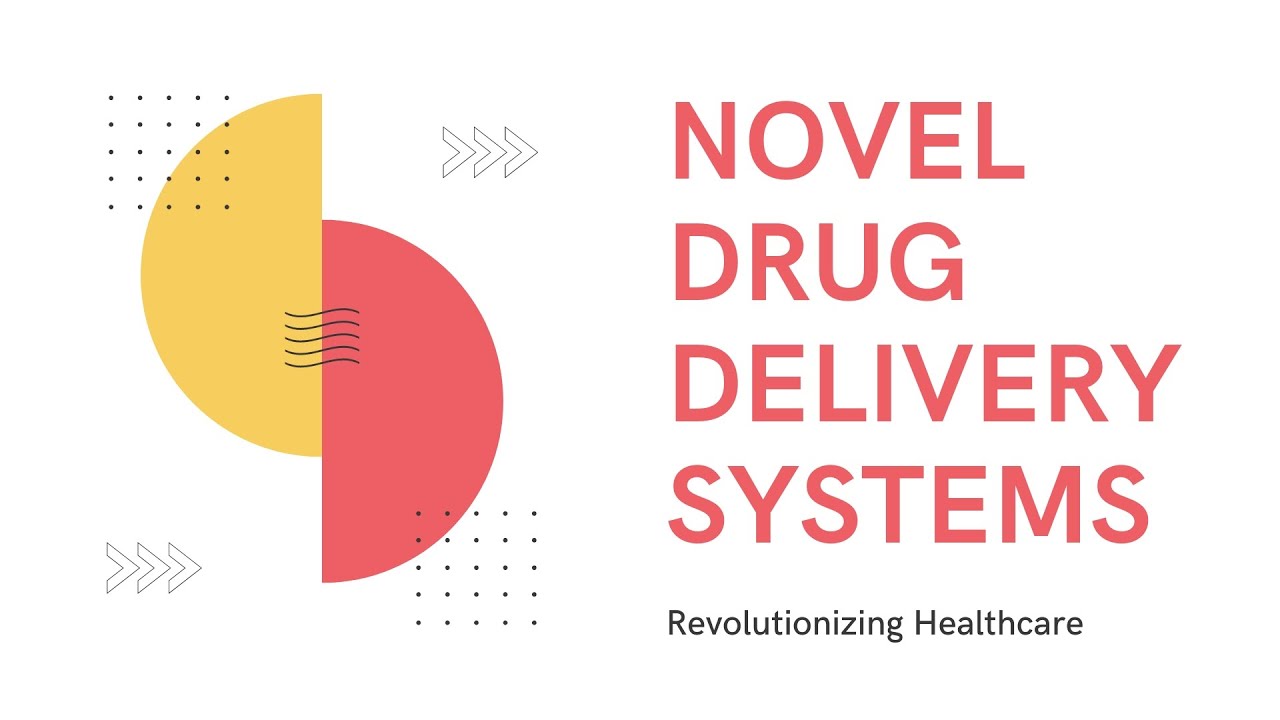
Introduction:
The landscape of healthcare is constantly evolving, with advancements in science and technology paving the way for innovative solutions. Among these breakthroughs, novel drug delivery systems have emerged as a transformative force, revolutionizing the field of medicine. In this blog, we embark on a journey through the world of novel drug delivery, exploring its significance, the science behind it, and the myriad ways in which it is reshaping patient care.
Traditional Drug Delivery vs. Novel Approaches:
Traditionally, drugs have been administered through conventional methods such as oral tablets, injections, or topical applications. While effective, these methods often face challenges, including inconsistent drug release, low bioavailability, and potential side effects. Novel drug delivery systems aim to overcome these limitations by providing targeted and controlled release of therapeutic agents, optimizing their efficacy while minimizing adverse effects.
The Science Behind Novel Drug Delivery Systems:
Nanotechnology: Nanotechnology has emerged as a game-changer in drug delivery. Nanoparticles, typically in the range of 1-100 nanometers, allow for precise control over drug release. Liposomes, micelles, and polymeric nanoparticles are examples of nanocarriers that enhance drug solubility, improve bioavailability, and enable targeted delivery to specific tissues or cells.
Understanding Nanotechnology:
Nanoscale Dimensions: At the nanoscale, materials exhibit unique properties and behaviors compared to their macroscopic counterparts. Quantum effects become more pronounced, and surface-to-volume ratios play a pivotal role, leading to altered electrical, optical, and chemical characteristics.
Interdisciplinary Nature: Nanotechnology is inherently interdisciplinary, drawing principles from physics, chemistry, biology, and engineering. The convergence of these fields enables researchers to design and manipulate materials at the atomic and molecular levels.
Bottom-Up vs. Top-Down Approaches: Nanofabrication techniques can be broadly categorized into bottom-up and top-down approaches. Bottom-up methods involve building structures atom by atom, while top-down methods involve reducing larger structures to the nanoscale. Both approaches contribute to the versatility of nanotechnology.
Applications Across Industries:
Medicine and Healthcare: Nanotechnology is revolutionizing medicine with applications in drug delivery, imaging, and diagnostics. Nanoparticles can be engineered to deliver drugs with precision, target specific cells, and enhance medical imaging techniques for accurate diagnosis.
Electronics and Computing: In the realm of electronics, nanotechnology has led to the development of smaller, more efficient devices. Nanoscale transistors, memory devices, and quantum dots are pushing the boundaries of computational capabilities.
Energy and Environment: Nanotechnology is playing a pivotal role in addressing energy challenges. Nanomaterials are being utilized for more efficient solar cells, energy storage devices, and catalysis for environmental remediation.
Materials Science: Nanomaterials exhibit extraordinary mechanical, electrical, and thermal properties. This has implications for the development of advanced materials, including super-strong nanocomposites, lightweight materials, and high-performance coatings.
Agriculture and Food Industry: Nanotechnology is contributing to sustainable agriculture with innovations such as nanopesticides for targeted crop protection, smart delivery systems for nutrients, and nanosensors for food safety and quality monitoring.
Breakthroughs in Nanotechnology:
Carbon Nanotubes: Carbon nanotubes, cylindrical structures of carbon atoms, are hailed for their exceptional strength, thermal conductivity, and electrical properties. They find applications in electronics, materials science, and even potential roles in space elevators.
Quantum Dots: Quantum dots are semiconductor nanoparticles that emit specific colors of light based on their size. These tiny structures have applications in medical imaging, display technologies, and solar cells.
Nanomedicine: Nanomedicine is advancing diagnostics and treatment options. Theranostic nanoparticles can simultaneously diagnose and treat diseases, while targeted drug delivery systems minimize side effects and improve therapeutic outcomes.
DNA Nanotechnology: Leveraging the unique properties of DNA, researchers are using DNA nanotechnology to create nanoscale structures with precision. This has implications in drug delivery, molecular computing, and nanoscale robotics.
Challenges and Concerns:
Toxicity and Safety: The potential toxicity of certain nanomaterials is a concern that necessitates thorough investigation. Understanding the interactions of nanoparticles with biological systems is crucial for safe and responsible use.
Ethical Considerations: Ethical considerations surround the use of nanotechnology in various fields, particularly in medicine and genetics. Discussions on privacy, consent, and potential misuse of nanotechnological advancements are ongoing.
Regulation and Standardization: As nanotechnology applications proliferate, the need for effective regulation and standardization becomes paramount. Establishing guidelines for the safe development and use of nanomaterials is an ongoing challenge.
Societal Impacts: The societal impacts of widespread nanotechnology adoption, including potential job displacement, changes in manufacturing processes, and access to advanced technologies, require careful consideration and planning.
Future Horizons:
Nanoelectronics and Quantum Computing: Nanotechnology is expected to play a pivotal role in the development of nanoelectronics and quantum computing. The manipulation of individual atoms and quantum states holds the promise of unprecedented computational power.
Nanorobotics and Medicine: The convergence of nanotechnology and robotics is giving rise to the field of nanorobotics. In medicine, nanorobots could be designed for targeted drug delivery, surgery at the cellular level, and even monitoring health from within the body.
Environmental Solutions: Nanotechnology offers innovative solutions for environmental challenges. Nanomaterials can be employed for efficient water purification, air filtration, and remediation of polluted sites, contributing to sustainable environmental practices.
Navigating the Nano Frontier
In conclusion, nanotechnology represents a frontier of scientific exploration with vast potential for transformative applications. As researchers continue to unlock the secrets of the nanoscale world, the convergence of nanotechnology with other cutting-edge fields is paving the way for unprecedented innovations. Navigating the challenges and ethical considerations associated with nanotechnology will be crucial in harnessing its full potential for the betterment of humanity. The journey into the nano frontier is both exciting and challenging, offering a glimpse into a future where the infinitesimally small shapes the course of scientific discovery and technological progress.
Microencapsulation: Microencapsulation involves enclosing drugs within tiny capsules, protecting them from degradation and facilitating controlled release. This technique is particularly useful for drugs sensitive to gastric acidity or those requiring prolonged action.
Microencapsulation: Unveiling the Tiny World of Controlled Release
Microencapsulation, a fascinating technology at the intersection of chemistry, engineering, and pharmaceuticals, has emerged as a powerful tool for controlled release and targeted delivery of substances. This innovative technique involves enclosing tiny particles or droplets within a protective coating, opening up a realm of possibilities in diverse industries. In this exploration, we delve into the intricate world of microencapsulation, examining its principles, methods, applications, and the significant impact it has across various fields.
Understanding Microencapsulation:
Definition: Microencapsulation is a process wherein tiny particles or droplets, often in the micrometer range, are coated or enclosed within a protective material. This protective coating, known as the microcapsule, serves various purposes, such as controlling release, improving stability, and facilitating targeted delivery.
Core Materials: The core materials that undergo microencapsulation can vary widely, including pharmaceuticals, flavors, fragrances, enzymes, and even living cells. The choice of core material depends on the intended application and desired outcomes.
Protective Coating: The protective coating is typically composed of polymers, proteins, lipids, or a combination of these materials. The selection of the coating material influences the release kinetics, stability, and compatibility with the core substance.
Methods of Microencapsulation:
Spray Drying: Spray drying involves atomizing a solution containing the core material and coating material into tiny droplets, which are then dried to form microcapsules. This method is suitable for heat-sensitive substances.
Coacervation: Coacervation is a process where a polymer-rich phase is formed around the core material through phase separation. This method is widely used for encapsulating pharmaceuticals, fragrances, and agricultural chemicals.
Fluidized Bed Coating: In fluidized bed coating, the core material is suspended in a fluidized bed, and the coating material is sprayed onto the particles. This method is known for producing uniform and high-quality microcapsules.
Emulsion Techniques: Emulsion-based methods involve creating an emulsion of the core material in a coating solution. Depending on the type of emulsion (water-in-oil or oil-in-water), different types of microcapsules can be produced.
Applications of Microencapsulation:
Pharmaceutical Industry: Microencapsulation is extensively used in pharmaceuticals for controlled drug release. This technology enhances the bioavailability of drugs, reduces side effects, and allows for targeted delivery to specific tissues or organs.
Food and Beverage: In the food industry, microencapsulation is employed to encapsulate flavors, fragrances, vitamins, and other sensitive ingredients. This ensures better stability, prolonged shelf life, and controlled release upon consumption.
Agriculture: Microencapsulation finds applications in agriculture for the controlled release of fertilizers, pesticides, and plant growth regulators. This improves the efficiency of agrochemicals while minimizing environmental impact.
Cosmetics and Personal Care: Cosmetics and personal care products utilize microencapsulation for the encapsulation of active ingredients such as vitamins, antioxidants, and skin-conditioning agents. This enhances the stability and efficacy of these ingredients.
Textiles: Microencapsulation is employed in textiles for various purposes, including the controlled release of fragrances, antimicrobial agents, and phase-change materials for improved comfort and functionality.
Advantages of Microencapsulation:
Controlled Release: One of the primary advantages of microencapsulation is the ability to achieve controlled and sustained release of the core material over an extended period. This is particularly beneficial in drug delivery and agricultural applications.
Protection of Core Material: The protective coating shields the core material from external factors such as light, oxygen, and moisture, thereby improving stability and preserving the integrity of sensitive substances.
Enhanced Bioavailability: In pharmaceuticals, microencapsulation can improve the bioavailability of drugs by overcoming solubility and absorption challenges. This ensures that a higher proportion of the drug reaches its target site.
Taste and Odor Masking: In the food and pharmaceutical industries, microencapsulation is employed to mask undesirable tastes or odors associated with certain ingredients, enhancing the overall acceptability of the product.
Versatility: Microencapsulation is a versatile technology that can be adapted to various core and coating materials, making it applicable across a wide range of industries and diverse substances.
Challenges and Considerations:
Cost: The cost of microencapsulation processes, including equipment, materials, and labor, can be a consideration. Balancing the benefits against the economic feasibility is crucial in industrial applications.
Scalability: Scaling up microencapsulation processes from laboratory-scale to industrial-scale production can pose challenges. Ensuring reproducibility and maintaining product quality at larger scales require careful optimization.
Uniformity and Consistency: Achieving uniformity in microcapsule size and coating thickness is critical for consistent performance. Variability in these parameters may impact the controlled release and efficacy of the microencapsulated material.
Biocompatibility: In pharmaceutical and medical applications, ensuring the biocompatibility of the microcapsule materials is essential. Compatibility with biological systems and absence of adverse reactions are paramount considerations.
Future Perspectives:
The future of microencapsulation holds exciting possibilities. Ongoing research aims to enhance the precision and efficiency of microencapsulation techniques, exploring new materials and methodologies. The integration of microencapsulation with emerging technologies, such as nanotechnology and 3D printing, opens avenues for even more sophisticated applications.
Unlocking Potential in Tiny Spheres
In conclusion, microencapsulation stands as a testament to the ingenuity of scientists and engineers in harnessing the power of the minuscule. Whether encapsulating pharmaceuticals for targeted drug delivery or preserving the freshness of flavors in food, this technology has far-reaching implications. As advancements continue to unfold, the tiny spheres of microencapsulation hold the potential to reshape industries, improve healthcare, and elevate the quality of products we encounter in our daily lives. The journey into the world of controlled release is an ongoing exploration, unlocking the potential encapsulated within these minuscule spheres.
Implantable Drug Delivery Systems: Implantable devices, such as drug-eluting implants or microchips, provide sustained and controlled release of drugs over an extended period. These devices are often used for chronic conditions, offering a more patient-friendly alternative to frequent administrations.
Inhalation Systems: Inhalation drug delivery is effective for treating respiratory conditions. Novel inhalation systems, including dry powder inhalers and nebulizers, ensure precise dosing and targeted delivery to the lungs, enhancing the therapeutic impact of respiratory medications.
Advantages of Novel Drug Delivery Systems:
Enhanced Bioavailability: Novel drug delivery systems often improve the bioavailability of drugs by optimizing their absorption and distribution in the body. This is particularly beneficial for drugs with low solubility or those prone to rapid metabolism.
Targeted Drug Delivery: Precision is a hallmark of novel drug delivery. Targeted delivery systems allow drugs to be directed specifically to the site of action, minimizing off-target effects and enhancing therapeutic efficacy.
Controlled Release: Controlled release mechanisms enable a steady and sustained delivery of drugs over time. This not only improves patient compliance but also reduces the frequency of administrations and minimizes fluctuations in drug levels.
Reduced Side Effects: By minimizing exposure of healthy tissues to therapeutic agents, novel drug delivery systems help mitigate adverse effects, enhancing the overall safety profile of medications.
Personalized Medicine: The advent of novel drug delivery aligns with the principles of personalized medicine. Tailoring drug formulations to individual patient characteristics allows for optimized treatment outcomes and improved quality of care.
Applications of Novel Drug Delivery Systems:
Cancer Therapy: Nanoparticle-based drug delivery systems show promise in cancer therapy. These systems can enhance the delivery of chemotherapeutic agents to tumor sites, improving treatment efficacy while reducing damage to healthy tissues.
Diabetes Management: Implantable devices and smart insulin delivery systems offer innovative solutions for diabetes management. These technologies aim to provide controlled insulin release, mimicking the physiological insulin secretion pattern.
Neurological Disorders: The blood-brain barrier poses a challenge in treating neurological disorders. Novel drug delivery systems, including nanoparticles and liposomes, are designed to overcome this barrier, opening new possibilities for the treatment of conditions like Alzheimer’s disease.
Infectious Diseases: Targeted drug delivery is crucial in the fight against infectious diseases. Nanocarriers can improve the delivery of antimicrobial agents to specific infection sites, optimizing treatment outcomes and reducing the risk of resistance.
Chronic Pain Management: Implantable devices and transdermal patches provide novel approaches to chronic pain management. These systems offer controlled release of pain medications, ensuring sustained relief with minimal side effects.
Challenges and Considerations:
Biocompatibility: Ensuring the biocompatibility of novel drug delivery systems is paramount. Compatibility with biological tissues and minimal risk of immune reactions are critical considerations in their design.
Manufacturing Complexity: The production of novel drug delivery systems can be complex and may require specialized manufacturing processes. Ensuring scalability and reproducibility is a challenge that researchers and manufacturers must address.
Regulatory Approval: Obtaining regulatory approval for novel drug delivery systems involves rigorous evaluation of safety, efficacy, and quality. Meeting regulatory standards and demonstrating the benefits of these systems are crucial steps in their widespread adoption.
Cost Considerations: The development and implementation of novel drug delivery systems may come with increased costs. Balancing the potential benefits against economic considerations is an ongoing challenge in the healthcare industry.
Future Perspectives:
The future of novel drug delivery systems holds immense promise. As research continues to unravel the complexities of drug formulations and delivery mechanisms, we can anticipate even more sophisticated approaches. The integration of smart technologies, such as sensors and real-time monitoring, may further enhance the precision and effectiveness of drug delivery.
Conclusion: Transforming Healthcare through Innovation
In conclusion, novel drug delivery systems represent a paradigm shift in healthcare, offering solutions to longstanding challenges in drug administration. From nanoparticles to implantable devices, these systems are ushering in a new era of precision medicine, where treatments are tailored to individual needs. As researchers, healthcare professionals, and industry leaders continue to collaborate and innovate, the impact of novel drug delivery systems on patient outcomes and the overall healthcare landscape is poised to be profound. We stand at the cusp of a healthcare revolution, where the convergence of science, technology, and patient-centric care is reshaping the way we approach and deliver therapeutic interventions.
Nanotechnology, the manipulation of matter on an atomic or molecular scale, has emerged as a revolutionary field with transformative implications across diverse industries. Operating at the nanoscale, where dimensions are measured in nanometers (one billionth of a meter), nanotechnology offers unprecedented control over materials and structures. In this exploration, we delve into the fascinating world of nanotech, examining its principles, applications, and the potential it holds for shaping the future.
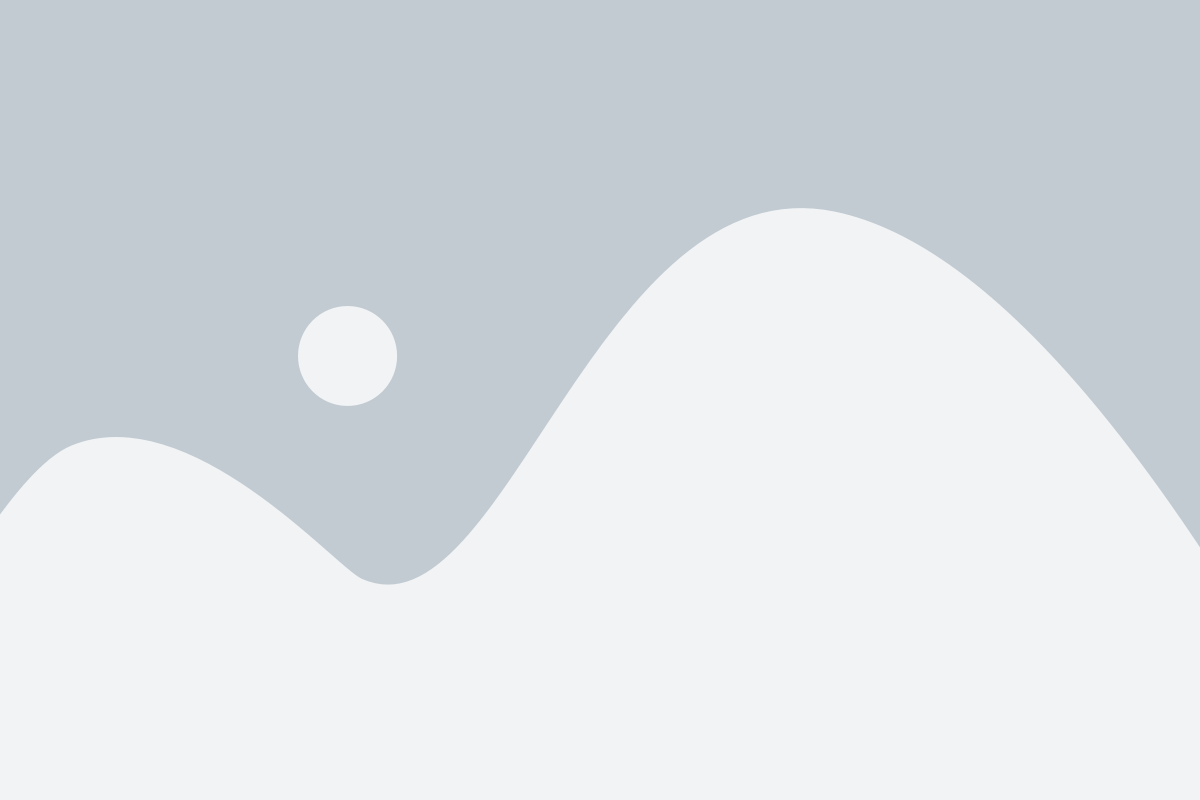
Dr. mamta
Geeta University, Panipat
Related Posts
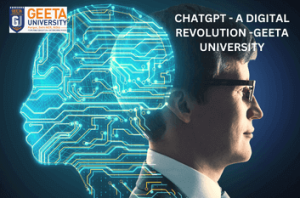
CHATGPT – A DIGITAL REVOLUTION -Geeta University
CHATGPT – A DIGITAL REVOLUTION –Geeta University As a language model developed by OpenAI, ChatGPT has revolutionized the world of communication and information exchange. With

Career Prospects and Scope of Computer Science Engineering – Geeta University
Computer Science Engineering, also known as Computer Science Engineering, is without a doubt one of the most sought after engineering specializations. B.Tech in CSE is
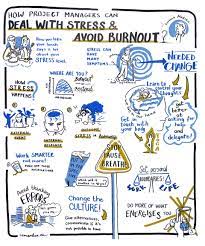
Stress management for students leaders
Stress management for students leaders The specific hurdles that come with combining academic responsibilities, extracurricular commitments, and leadership roles are not something that student leaders