Introduction
In the realm of construction, concrete stands as the cornerstone material, quite literally bearing the weight of infrastructure worldwide. However, traditional concrete comes with its set of limitations – susceptibility to cracking, erosion, and environmental degradation, to name a few. Enter nanotechnology, a field revolutionizing materials science, including the very concrete beneath our feet. In this blog, we delve into the promising realm of nanotechnology in concrete and its transformative potential in construction.
Understanding Nanotechnology in Concrete:
Nanotechnology in concrete involves the incorporation of nanomaterials, particles with dimensions less than 100 nanometers, to enhance its properties. These nanomaterials can be derived from various sources, including nanosilica, carbon nanotubes, graphene, and nanoclays, each offering unique benefits to the concrete matrix. Nanotechnology operates at the nanoscale, manipulating materials at dimensions smaller than 100 nanometers. When applied to concrete, nanotechnology introduces nanomaterials such as nanoparticles, nanofibers, and nanotubes to enhance its properties. These materials possess extraordinary characteristics, including high strength, durability, and reactivity, making them ideal candidates for concrete improvement.
Enhanced Strength and Durability:
One of the primary advantages of incorporating nanotechnology into concrete is the significant enhancement of its mechanical properties. Nanoparticles like silica fume, titanium dioxide, and carbon nanotubes disperse uniformly within the concrete matrix, reinforcing its structure and increasing compressive strength. This augmented strength not only improves the load-bearing capacity of structures but also extends their lifespan by reducing the likelihood of cracks and structural failures.
Self-Healing Capabilities:
Cracks are a common issue in conventional concrete, compromising its integrity and longevity. Nanotechnology offers a promising solution through self-healing concrete. Microcapsules filled with healing agents, such as polymers or bacteria, are embedded in the concrete mix. When cracks form, these capsules rupture, releasing the healing agent that fills the gaps, effectively repairing the concrete. This innovative approach ensures the continuous functionality of structures and reduces the need for frequent maintenance.
Environmental Sustainability of nano enhanced concrete:
Beyond performance improvements, nanotechnology in concrete contributes to environmental sustainability. By enhancing the durability and longevity of structures, it reduces the need for frequent repairs and replacements, thus lowering material consumption and associated carbon emissions. Additionally, the use of nanomaterials allows for the utilization of waste by-products, such as fly ash and slag, in concrete production, mitigating the environmental impact of traditional manufacturing processes.
Environmental stability refers to the ability of a material, product, or system to withstand and maintain its performance and integrity when exposed to various environmental conditions over time. In the context of nanotechnology in concrete, ensuring environmental stability is crucial for long-term durability and sustainability of concrete structures. Let’s explore how environmental stability is achieved and its significance in the context of nano-enhanced concrete.
Factors Affecting Environmental Stability
Several factors influence the environmental stability of nano-enhanced concrete:
1. Exposure Conditions: Concrete structures are subjected to a wide range of environmental factors, including temperature variations, moisture levels, chemical exposure, and mechanical loading. Nano-enhanced concrete must be designed to withstand these conditions without compromising its performance.
2. Material Composition: The selection and incorporation of nanomaterials play a significant role in determining the environmental stability of concrete. Nanoparticles with high chemical and physical stability are preferred to ensure long-term performance.
3. Interaction with Surrounding Environment: Nano-enhanced concrete interacts with its surrounding environment, including soil, water, and air. Understanding these interactions and their potential effects on concrete properties is essential for predicting long-term performance.
Significance of Environmental Stability
1. Durability: Environmental stability is closely linked to the durability of concrete structures. Nano-enhanced concrete with high environmental stability exhibits improved resistance to degradation mechanisms such as corrosion, alkali-silica reaction, and sulfate attack, leading to extended service life.
2. Sustainability: Sustainable construction practices aim to minimize environmental impact throughout the lifecycle of structures. Environmental stability ensures that nano-enhanced concrete maintains its performance over time, reducing the need for frequent repairs or replacements and conserving resources.
3. Cost-Effectiveness: Enhancing the environmental stability of concrete structures results in long-term cost savings by minimizing maintenance and repair costs. Nano-enhanced concrete with superior durability reduces lifecycle costs and improves the overall economic viability of construction projects.
4. Safety: Environmental stability contributes to the safety of concrete structures by maintaining structural integrity and preventing potential hazards associated with deterioration or failure.
Strategies for Achieving Environmental Stability
1. Material Selection: Careful selection of nanomaterials based on their chemical and physical properties is essential for achieving environmental stability. Nanoparticles with high chemical resistance and stability in different environmental conditions are preferred.
2. Optimized Mix Design: Incorporating nano-enhanced materials into the concrete mix design in appropriate proportions ensures uniform dispersion and compatibility with other components, enhancing overall environmental stability.
3. Surface Protection: Surface treatments and coatings can provide an additional layer of protection against environmental factors, further enhancing the stability of nano-enhanced concrete.
4. Quality Control: Rigorous quality control measures during material production, mixing, and placement are essential to ensure the environmental stability of nano-enhanced concrete structures.
Environmental stability is a critical aspect of nano-enhanced concrete, ensuring long-term durability, sustainability, and safety of concrete structures. By understanding the factors influencing environmental stability and implementing appropriate strategies, engineers and researchers can develop nano-enhanced concrete solutions that withstand the challenges of the built environment while contributing to a more sustainable and resilient infrastructure.
Applications in Construction:
The applications of nanotechnology in concrete span across various sectors of construction, from residential buildings to infrastructure projects. High-performance concrete infused with nanoparticles finds application in bridges, tunnels, and high-rise buildings, where structural integrity is paramount. Self-healing concrete is particularly beneficial in critical infrastructure like nuclear power plants and offshore structures, where maintenance access is limited, and structural failures can have catastrophic consequences.
Nanotechnology has made significant strides in revolutionizing various aspects of the construction industry. By manipulating materials at the nanoscale, researchers and engineers have developed innovative solutions to enhance the performance, durability, and sustainability of construction materials and structures. Here are some notable applications of nanotechnology in construction:
1. Nano-Enhanced Concrete
Nanotechnology has been extensively applied to improve the properties of concrete, the most widely used construction material. Nano-sized additives such as silica nanoparticles, carbon nanotubes, and graphene oxide are incorporated into concrete mixes to enhance mechanical strength, durability, and resistance to environmental degradation. Nano-enhanced concrete exhibits higher compressive strength, reduced permeability, and enhanced resistance to corrosion and cracking, leading to longer service life and reduced maintenance needs.
2. Self-Cleaning and Photocatalytic Surfaces
Nanotechnology has enabled the development of self-cleaning and photocatalytic coatings for building facades and surfaces. Nanostructured titanium dioxide (TiO2) coatings are applied to building exteriors to harness photocatalytic properties, enabling the breakdown of organic pollutants and the self-cleaning of surfaces through the action of sunlight. These coatings help maintain the aesthetic appearance of buildings and reduce the need for manual cleaning and maintenance.
3. Nano-Insulation Materials
Nanotechnology has contributed to the development of advanced insulation materials with superior thermal performance. Nanoscale additives such as aerogels, nanofoams, and nanoporous materials are incorporated into insulation products to enhance thermal resistance while minimizing material thickness. Nano-insulation materials offer improved energy efficiency, reduce heating and cooling costs, and contribute to sustainable building practices by lowering energy consumption and greenhouse gas emissions.
4. Nano-Coatings for Waterproofing and Corrosion Protection
Nanostructured coatings are applied to building surfaces, including concrete, steel, and masonry, to provide waterproofing and corrosion protection. These coatings form a thin, protective layer that repels water and inhibits the penetration of corrosive agents, such as chloride ions, thereby extending the lifespan of structures in harsh environments. Nano-coatings offer a cost-effective solution for enhancing the durability and longevity of building materials while reducing maintenance requirements.
5. Nanotechnology in Smart Materials and Sensors
Nanotechnology plays a crucial role in the development of smart materials and sensors for structural health monitoring and diagnostics. Nanomaterial-based sensors embedded within concrete structures enable real-time monitoring of structural integrity, strain, temperature, and other parameters. These sensors provide valuable data for assessing structural health, detecting early signs of deterioration or damage, and implementing proactive maintenance strategies to prevent catastrophic failures.
6. Lightweight and High-Strength Nanocomposites
Nanotechnology has facilitated the development of lightweight and high-strength nanocomposite materials for various construction applications. Nano-reinforcements such as carbon nanotubes, nanofibers, and graphene are incorporated into polymer matrices to create lightweight composites with exceptional mechanical properties. These nanocomposites offer advantages such as high strength-to-weight ratio, enhanced impact resistance, and improved structural performance, making them ideal for aerospace, automotive, and infrastructure applications.
7. Nanotechnology in Sustainable Construction
Nanotechnology contributes to sustainable construction practices by enhancing the performance and environmental impact of building materials. Nano-additives enable the use of alternative and recycled materials in concrete mixes, reducing the reliance on virgin resources and minimizing waste. Additionally, nano-coatings and surface treatments improve the energy efficiency of buildings by reducing heat transfer and improving thermal insulation, thereby lowering energy consumption and carbon emissions.
Nanotechnology holds immense potential to transform the construction industry by offering innovative solutions to enhance the performance, durability, and sustainability of building materials and structures. From nano-enhanced concrete and self-cleaning surfaces to smart materials and sustainable nanocomposites, the applications of nanotechnology in construction continue to expand, driving advancements in building design, construction techniques, and environmental stewardship.
Challenges and Considerations:
While nanotechnology holds immense promise for revolutionizing concrete, several challenges and considerations need to be addressed. These include the cost-effectiveness of nanomaterials, potential health and environmental impacts during production and application, and the scalability of manufacturing processes. Additionally, further research is needed to optimize nanomaterial incorporation techniques and assess long-term performance under real-world conditions.
While nanotechnology offers promising opportunities in construction, it also presents several challenges that need to be addressed for its widespread adoption and successful implementation. These challenges span various aspects, including technological, economic, environmental, and regulatory considerations. Let’s explore some of the key challenges associated with the application of nanotechnology in construction:
1. Cost
Challenge: One of the primary challenges of integrating nanotechnology into construction materials is the high cost associated with nanomaterials and manufacturing processes. Nano-additives, such as nanoparticles and nanofibers, are often more expensive than traditional materials, which can significantly increase the overall cost of construction projects.
Solution: Research and development efforts are focused on optimizing production processes, scaling up manufacturing capabilities, and exploring cost-effective synthesis methods for nanomaterials. Additionally, the long-term benefits of enhanced performance and durability offered by nano-enhanced materials may justify the initial investment in certain applications.
2. Scaling Up Production
Challenge: The production of nanomaterials on a large scale presents challenges related to scalability, reproducibility, and quality control. Existing laboratory-scale synthesis methods may not be suitable for mass production, leading to limitations in meeting the demand for nano-enhanced construction materials.
Solution: Collaborative efforts between researchers, industry stakeholders, and government agencies are essential to develop scalable production techniques for nanomaterials. Investments in advanced manufacturing technologies and infrastructure can facilitate the commercialization of nano-enhanced construction materials.
3. Health and Safety
Challenge: Nanomaterials pose potential health and safety risks to workers involved in their production, handling, and application. Exposure to airborne nanoparticles may lead to respiratory problems, skin irritation, and other adverse health effects, highlighting the importance of implementing appropriate safety measures.
Solution: Strict adherence to occupational health and safety regulations, as well as the development of comprehensive risk assessment protocols, is crucial to mitigate the potential hazards associated with nanomaterials. Training programs, personal protective equipment (PPE), and engineering controls can help minimize exposure risks in construction settings.
4. Environmental Impact
Challenge: While nanotechnology offers opportunities to improve the environmental performance of construction materials, concerns exist regarding the environmental impact of nanomaterials throughout their lifecycle. This includes issues related to resource depletion, energy consumption, and end-of-life disposal of nano-enhanced products.
Solution: Life cycle assessments (LCAs) and environmental impact studies are essential for evaluating the overall environmental footprint of nano-enhanced construction materials. Sustainable sourcing of raw materials, eco-friendly production processes, and recycling/reuse strategies can help mitigate environmental impacts and promote responsible use of nanotechnology in construction.
5. Regulatory Framework
Challenge: The regulatory landscape for nanotechnology in construction is still evolving, with limited standardization and guidelines for the development and application of nano-enhanced materials. Uncertainties regarding regulatory requirements and compliance may hinder investment and innovation in this field.
Solution: Collaborative efforts between government agencies, industry associations, and research institutions are needed to establish robust regulatory frameworks for nanotechnology in construction. This includes defining safety standards, labeling requirements, and environmental regulations to ensure the responsible use and commercialization of nano-enhanced materials.
While nanotechnology holds immense promise for advancing construction materials and techniques, addressing the challenges outlined above is crucial for realizing its full potential. By overcoming barriers related to cost, scalability, health and safety, environmental impact, and regulatory compliance, the construction industry can harness the transformative power of nanotechnology to build safer, more sustainable, and resilient infrastructure for the future.
Conclusion:
Nanotechnology in concrete represents a paradigm shift in construction, offering solutions to traditional challenges while paving the way for more sustainable and resilient infrastructure. By harnessing the unique properties of nanomaterials, concrete can achieve unprecedented levels of strength, durability, and functionality. As research and development in this field continue to advance, the widespread adoption of nanotechnology in concrete holds the promise of shaping a more resilient, efficient, and environmentally conscious built environment for generations to come.
Related Posts
REVOLUTIONIZING CONSTRUCTION: THE IMPACT OF NANOTECHNOLOGY IN CONCRETE AND ENVIRONMENTAL SUSTAINABILITY
Introduction In the realm of construction, concrete stands as the cornerstone material, quite literally bearing the weight of infrastructure worldwide. However, traditional concrete comes with
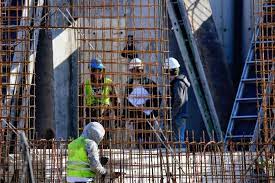
BUILDING A SUSTAINABLE FUTURE WITH BACTERIAL CONCRETE
Introduction: In the quest for sustainable construction materials, scientists and engineers are constantly exploring innovative solutions to address the challenges of environmental degradation and resource
FORENSIC SOMATOSCOPY AND SOMATOMETRY
Forensic Somatometry Forensic somatoscopy is a branch of forensic science that involves the examination and analysis of physical features and characteristics of the body of