Introduction
- May 27, 2024
- Geeta University
One kind of nanostructured material made up of scattered nanocrystals within a gel matrix is called a nanocrystal gel. By utilizing the special qualities of nanocrystals, these gels can produce materials with improved mechanical, optical, and electrical properties. Understanding the interactions between nanocrystals and the gel matrix,
as well as the ensuing properties and possible uses, is essential to comprehending the theory underlying nanocrystal gels. They have special qualities like high surface area, enhanced reactivity, and quantum confinement effects because of their small size. Organic polymers, inorganic materials, or a combination of the two can make up the matrix.
Gels are useful materials for drug delivery, wound dressings, tissue engineering, and 3D printing. These various applications require gels with different mechanical properties that can be easily tuned, also preferably excluding the use of chemical additives, which can be toxic or harmful to the body or environment.
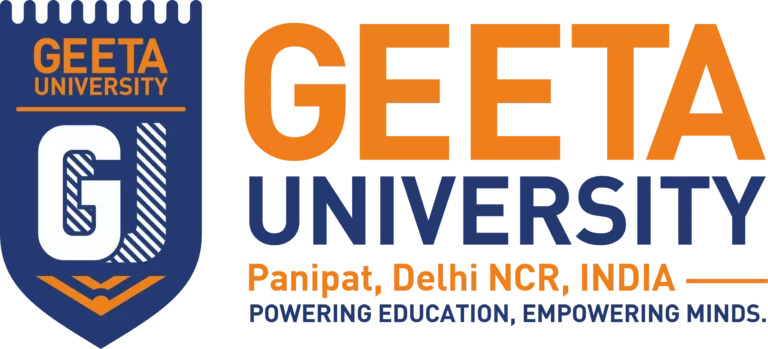
Admission Open 2024-2025
For Your bright Future
Structure based gels:
• Homogeneous Gels: These gels have a uniform distribution of nanocrystals throughout the matrix, providing consistent properties throughout the material.
• Core-Shell Structures: Nanocrystals can be encapsulated within a core-shell structure, where the core is the nanocrystal and the shell is the gel matrix. This configuration can protect the nanocrystals and enhance their functionality.
• Composite Gels: These gels contain multiple types of nanocrystals or a combination of nanocrystals and other functional materials, offering multifunctional properties.
Polysaccharide nanocrystals in the field of functional nanomaterials:
Polysaccharides belong to the class of molecules that are referred to as carbohydrates. Natural polymers that are widely distributed in nature as structural building blocks are called polysaccharides, and examples include cellulose and chitin. For fuel cells, other polysaccharides (like starch) offer stored solar energy in the form of sugar. Chitin, starch, and cellulose are examples of natural polysaccharides that have both crystalline and amorphous sections. Because of their high hydrolysis susceptibility, the amorphous regions may be eliminated under certain circumstances while maintaining the rigid crystalline regions. There are various techniques for removing the crystalline regions from the biomass. The material that has been recovered takes the shape of polysaccharide nanocrystals, most frequently in the form of chitin nanowhiskers, platelet-like starch nanocrystals, and rod-like cellulose nanocrystals (also known as nanocrystalline cellulose).
Due to the controllable and convenient treatment, acid hydrolysis is mostly utilized in the preparation of polysaccharide nanocrystals, although the conditions of hydrolysis during the extraction process (such as the type of acid, acid concentration, temperature, and duration) are different.
Some common methods include:
• enzymatic-mediated production of cellulose nanocrystals from recycled pulp:
• Ionic liquid preparation of chitin nanowhiskers or cellulose nanocrystals
• mechanical separation processes such as ceramic membrane filtration for isolating starch nanocrystals,
• sonochemical-assisted hydrolysis preparation of cellulose nanocrystals,
• Combined mechanical shearing, enzymatic and acid hydrolysis extraction of cellulose nanocrystals
Numerous studies have detailed the benefits of natural polysaccharide nanocrystals over inorganic nanoparticles, which primarily include their accessibility, biocompatibility, biodegradability, ease of processing, nanoscale effects, affordability, high specific strength and modulus, and relatively reactive surface.
Polysaccharide nanocrystals are highly useful in the field of materials science due to their exceptional qualities and traits. The majority of these studies concentrate on using polysaccharide nanocrystals to reinforce polymeric matrices through potential chemical and physical surface modification. The primary goal of this approach is to enhance the mechanical and thermal performances of nanocomposite materials. Several unique characteristics and benefits of polysaccharide nanocrystals include their high aspect ratio, stiffness, highly crystalline characteristics, active functional groups, and chiralnematic phase.
Physicochemical properties of polysaccharide Nanocrystals:
• Morphology and geometric dimensions
• Stiffness and crystalline character
• Thermal properties
• Dispersion of polysaccrides nanocrystals in solvent
• Rheological properties
• Liquid crystalline character
Common Types of Polysaccharide Nanocrystals
1. Cellulose Nanocrystals (CNCs):
• Derived from cellulose, the most abundant natural polymer found in the cell walls of plants.
• Known for their high strength, stiffness, and biodegradability.
• Applications: Reinforcement in composites, biomedical applications, barrier materials in packaging.
2. Chitin and Chitosan Nanocrystals:
• Chitin is found in the exoskeleton of crustaceans and insects, and chitosan is derived from chitin through deacetylation.
• Exhibits antimicrobial properties, biocompatibility, and biodegradability.
• Applications: Drug delivery systems, wound healing materials, water purification.
3. Starch Nanocrystals:
• Derived from starch, which is found in many plants such as corn, potatoes, and rice.
• Exhibits good film-forming properties, biodegradability, and non-toxicity.
• Applications: Food packaging, biodegradable plastics, controlled release of bioactive compounds.
4. Xylan Nanocrystals:
• Derived from xylan, a hemicellulose found in the cell walls of plants.
• Known for their ability to form hydrogels and good mechanical properties.
• Applications: Hydrogels for biomedical applications, food thickeners, and stabilizers.
Synthesis of Polysaccharide Nanocrystals
The synthesis of polysaccharide nanocrystals typically involves the breakdown of the amorphous regions of polysaccharides to isolate the crystalline regions.
Common methods include:
1. Acid Hydrolysis:
• A common method for producing cellulose nanocrystals involves treating cellulose fibers with strong acids like sulfuric or hydrochloric acid to remove amorphous regions, leaving behind the crystalline regions.
• The process parameters (e.g., temperature, acid concentration, and time) influence the size and properties of the resulting nanocrystals.
2. Enzymatic Hydrolysis:
• Uses specific enzymes to selectively break down amorphous regions of polysaccharides.
• Offers a more environmentally friendly approach compared to acid hydrolysis.
3. Mechanical Methods:
• High-shear homogenization, ultrasonication, and ball milling can break down polysaccharide fibers into nanocrystals.
• Often used in combination with chemical treatments to improve efficiency.
Properties of Polysaccharide Nanocrystals
1. Mechanical Properties:
• High tensile strength and stiffness due to their crystalline structure.
• Potential for reinforcing polymer composites to improve mechanical properties.
2. Biodegradability:
• Naturally derived and biodegradable, making them environmentally friendly alternatives to synthetic materials.
3. Surface Chemistry:
• Abundance of hydroxyl groups on their surface allows for chemical modification and functionalization.
• Can be tailored for specific applications by attaching functional groups or other molecules.
4. Optical Properties:
• Depending on the type and structure, they can exhibit unique optical properties, such as birefringence.
Applications
1. Biomedicine:
• Drug Delivery: Polysaccharide nanocrystals can be used to deliver drugs in a controlled manner due to their biocompatibility and ability to encapsulate bioactive compounds.
• Tissue Engineering: Used in scaffolds to support cell growth and tissue regeneration.
2. Food Industry:
• Edible Films and Coatings: Improve the mechanical and barrier properties of food packaging.
• Stabilizers: Enhance the texture and stability of food products.
3. Materials Science:
• Nanocomposites: Reinforcement in polymers to enhance mechanical properties and create lightweight, high-strength materials.
• Barrier Materials: Used in packaging to improve barrier properties against gases and moisture.
4. Environmental Applications:
• Water Purification: Used to remove contaminants due to their high surface area and functionalizable surface.
Future Aspects
1. Functionalization:
• Further development of methods to functionalize polysaccharide nanocrystals for specific applications, such as targeted drug delivery or specific interactions in composite materials.
2. Sustainable Production:
• Developing greener and more efficient synthesis methods to reduce environmental impact and make production more scalable.
3. Advanced Applications:
• Exploring new applications in electronics, photonics, and advanced materials where their unique properties can be leveraged.
Polysaccharide nanocrystals represent a versatile and sustainable class of nanomaterials with a wide range of potential applications, driven by their unique properties and the ability to modify their surface chemistry.
Inorganic polysaccharides are not common in nature as most polysaccharides are organic compounds consisting of long chains of monosaccharide units (sugars). However, in materials science and chemistry, there are inorganic polymers and networks that can exhibit properties similar to organic polysaccharides. These materials are often referred to as “inorganic polymers” and can include structures that mimic the long-chain, network-forming behavior of organic polysaccharides but are composed of inorganic elements.
Inorganic nanocrystal gels retain distinct properties of individual nanocrystals while offering tunable, network-structure-dependent characteristics.
Different mechanisms for assembling gels from colloidal nanocrystals including
• Controlled destabilization,
• Direct bridging,
• Depletion, as well as linking mediated by
• Coordination bonding or
• Dynamic covalent bonding,
we highlight how each impacts gel properties. These approaches use nanocrystal surface chemistry or the addition of small molecules to mediate inter-nanocrystal attractions. Each method offers advantages in terms of gel stability, reversibility, or tunability and presents new opportunities for the design of reconfigurable materials and fueled assemblies.
Inorganic Polymers
Inorganic polymers are materials with a backbone consisting of elements other than carbon. They can have repeating units that include elements like silicon, phosphorus, boron, nitrogen, and others. Some of the key types of inorganic polymers include:
1. Silicones (Polysiloxanes):
• Structure: Silicon-oxygen (Si-O) backbone with organic side groups attached to the silicon atoms.
• Properties: Flexibility, thermal stability, water repellency, and biocompatibility.
• Applications: Used in sealants, adhesives, medical devices, and lubricants.
2. Polyphosphazenes:
• Structure: Phosphorus-nitrogen (P-N) backbone with organic or inorganic side groups attached to the phosphorus atoms.
• Properties: Thermal stability, flame resistance, and flexibility.
• Applications: Used in specialty elastomers, membranes, and biomedical applications.
3. Polysilanes:
• Structure: Silicon-silicon (Si-Si) backbone with organic side groups attached to the silicon atoms.
• Properties: Unique electronic properties, photoconductivity.
• Applications: Used in electronic and optoelectronic devices.
Inorganic Networks and Gels
In addition to inorganic polymers, there are inorganic networks and gels that can form three-dimensional structures similar to those of organic polysaccharides:
1. Silica Gels:
• Structure: Three-dimensional network of silicon dioxide (SiO₂).
• Properties: High surface area, porous structure, thermal stability.
• Applications: Used in chromatography, desiccants, and as catalyst supports.
2. Zeolites:
• Structure: Crystalline aluminosilicate frameworks with microporous structure.
• Properties: High surface area, ion-exchange capacity, catalytic activity.
• Applications: Used in catalysis, adsorption, and ion-exchange processes.
3. Alumina Gels:
• Structure: Three-dimensional network of aluminum oxide (Al₂O₃).
• Properties: High surface area, thermal stability.
• Applications: Used as adsorbents, catalyst supports, and in chromatography.
Characteristics and Applications
Inorganic polysaccharide-like materials often exhibit unique properties such as:
• Thermal Stability: Resistance to high temperatures compared to organic counterparts.
• Chemical Resistance: Stability in harsh chemical environments.
• Mechanical Properties: High strength and durability.
• Functional Versatility: Ability to incorporate various functional groups and tailor properties for specific applications.
These properties make them suitable for a wide range of applications, including:
• Catalysis: As supports or active materials in catalytic reactions.
• Environmental Remediation: In water and air purification processes.
• Biomedical Applications: In drug delivery, medical devices, and tissue engineering.
• Advanced Materials: In electronic, photonic, and structural applications.
Future aspects
Research in the field of inorganic polymers and networks continues to expand, focusing on:
• Synthesis Techniques: Developing new methods to synthesize and process these materials with controlled properties.
• Hybrid Materials: Combining organic and inorganic components to create hybrid materials with enhanced or novel properties.
• Functionalization: Exploring ways to functionalize these materials to tailor their properties for specific applications.
• Sustainable Materials: Investigating environmentally friendly and sustainable production methods for these materials.
Inorganic polysaccharide-like materials, although not common in the same sense as organic polysaccharides, represent a versatile and valuable class of materials with a wide range of applications driven by their unique properties and structural characteristics.
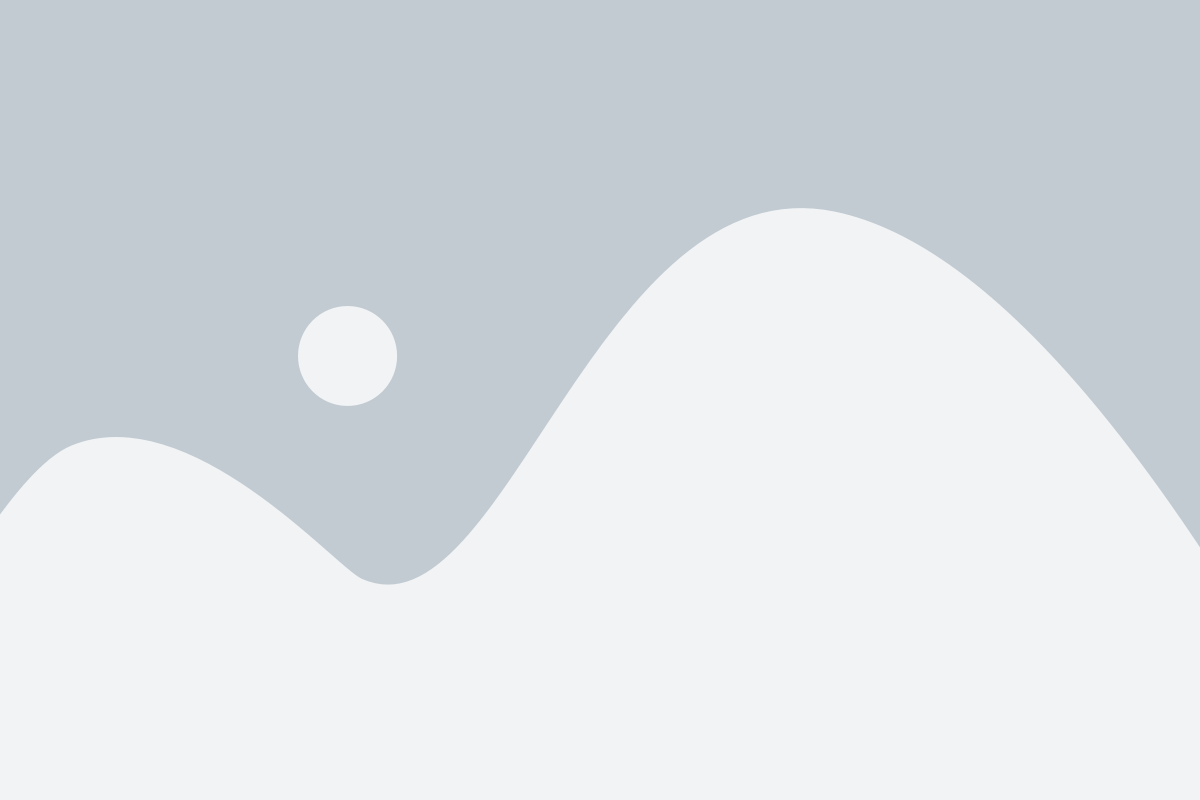
Ms. Manita
Assistant Professor
Geeta Institute of Pharmacy
Tags
Related Posts
Alternate Methods for Reducing Cardiovascular Risk
Reducing cardiovascular risk involves a combination of lifestyle changes, dietary adjustments, and, in some cases, medication. Here are several effective methods to help lower your risk of cardiovascular disease: Lifestyle Changes 1. Quit Smoking: o Smoking is a major risk