Microbial Synthesis of Nanoparticles
Uncategorized Uncategorized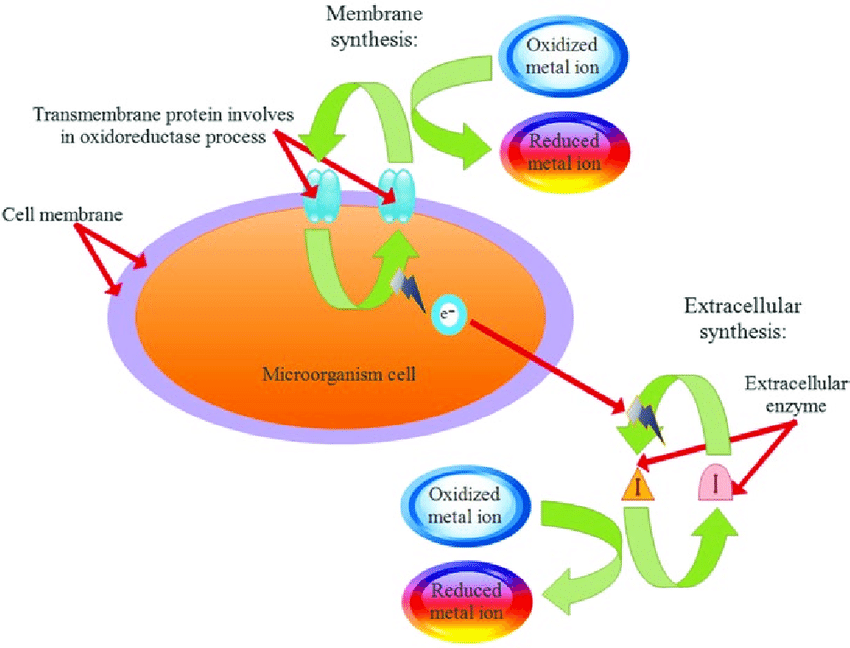
INTRODUCTION:
Nanoparticles, which are demarcated as particles with as a minimum one measurement measuring hundred nanometres or fewer, have gained a lot of consideration because of their distinctive and alluring characteristics as well as their beneficial applications compared to their larger counterparts. These particles, often referred to as particulate matter, are the molecular components of Nanotechnology. They can occur naturally or be produced by human activity and array in dimensions from 1 to 100 nanometres. Their incredibly small size bestows unique material properties. Different types of nanoparticles can be manufactured by employing a diversity of procedures, including physical, biotic, hybrid ones and chemical.
Despite the widespread use of physical and chemical techniques for nanoparticle manufacturing, the presence of harmful substances places serious restrictions, particularly in biomedical applications and clinical settings. Hence, the pursuit of dependable, innocuous and eco-friendly techniques for nanoparticle synthesis is of paramount significance in order to broaden their biomedical applications. One viable approach to accomplish this objective is the utilization of microorganisms in production of nanoparticles. These innovations are thought to have the potential to benefit industries including medicine research and water purification. They involve the production of materials and their nanoscale manipulation.
In comparison to chemically created nanoparticles, those made using a biogenic enzymatic process have a number of significant advantages. Chemical techniques are complicated, obsolete, expensive and ineffective, despite the fact that they can rapidly manufacture an enormous quantity of nanoparticles with appropriate proportions and shape. In addition, chemical operations produce toxic waste which is dangerous for both the atmosphere and people’s wellbeing.
Enzymatic techniques, on the other hand, eliminate off the requirement for costly chemicals, conforming to a more sustainable and “green” method. In addition to using less energy and being easier on the environment, biological procedures are preferable to chemical ones. It should be noted that the “biogenic” technique benefits from the fact that many bacteria can survive in a variety of environments with different pressure, pH, and temperature. Nanoparticles generated via biogenic procedures display enhanced catalytic activity, feature an increased explicit surface-area, furthermore facilitate enhanced quality contact amongst the enzyme and the metal salt owing to the bacterial carrier matrix. The biogenesis of nanoparticles involves the extraction of target ions from the environment by microorganisms, followed by the transfiguration of these metal ions into elemental metals by enzymes engendered by the cell’s metabolic processes.
Based on how the nanoparticles are created, there are two basic forms of nanoparticle synthesis: external and intracellular. In the intracellular method, metallic ions are introduced into microbial cells in the presence of enzymes, are transfigured into nanoparticles. Alternatively, metal ions are confined on cell surfaces during extracellular creation of nanoparticles, where they are abridged into nanoparticles in the existence of enzymes.
Nanoparticles created via biosynthesis have diverse applications, including serving as precise drug delivery vehicles, cancer treatments, tools for gene therapy, and aids in DNA analysis. They are also effective as antibacterial agents, biosensors, accelerators of reaction rates in diverse processes, contributors to separation science, and contributors to the advancement of MRI applications. This chapter explores into the mechanisms and factors influencing microbial nanoparticle synthesis, as well as its diverse applications in medicine, environmental remediation, and material science.
2. MECHANISMS OF MICROBIAL NANOPARTICLE SYNTHESIS
Nanoparticle formation is accomplished by various microbes via various processes. Microbial fusion of nanoparticles primarily encompasses the lessening of metal ions extant in the environment. This reduction can occur extracellularly or intracellularly, mediated by enzymes, biomolecules and metabolic pathway. Intracellular synthesis often leads to the development of well-defined nanoparticles due to precise control over reaction conditions within the microbial cell. In contrast, extracellular synthesis occurs through the interaction of microbial secretions with metal ions, resulting in nanoparticle formation. Nanoparticles of gold and silver were observed to be formed on the surface of the mycelium of Verticillium sp. and not in the solution. It was the basis of all the ongoing research.
The development of these metallic nanoparticles is attributed to the genetic and proteomic response of metallophillic microorganisms to a noxious environment.
Kalishwaralal and his team postulated that the generation of Ag nanoparticles within B. licheniformis is mediated by the nitrate reductase enzyme. This enzyme, activated by nitrate ions, enables the conversion of Ag ions into metallic Ag. The likely mechanism for decreasing silver ions involves an enzymatic metal reduction process in which electrons are transported to facilitate the reduction. NADH-dependent nitrate reductase and NADH enzymes play crucial roles in this biosynthetic process for metal nanoparticles. B. licheniformis is recognized for its secretion of NADH and NADH-dependent enzymes, notably nitrate reductase. These constituents are believed to contribute to the bio-reduction of Ag+ into Ag0, thereby facilitating the proffering of silver nanoparticles.
In order to counter these lethal properties for the subsistence of these microorganisms, they have evolved the hereditary and proteomic riposte to stringently control metallic homeostasis. These microorganisms haven a numeral of metal resistance genes which enables cell detoxification via numerous mechanisms i.e., efflux, reductive precipitation etc. Therefore, these bacteria can flourish in the environments having high concentrations of hefty metal ions.
SYNTHESIS:
The joining of nanoparticles by means of microbial procedures has arisen as an globally approachable and enduring alternative to conventional chemical techniques. Microorganisms possess inherent capabilities to condense metallic ions and generate nanoparticles of controlled sizes, shapes and compositions.
Metallic Nanoparticles and Gold Nanoparticles
The use of gold nanoparticles (AuNPs) to colour glasses for decorative purposes dates back to the early Roman era, and they have since gained important historical significance in the field of chemistry. AuNPs were even used to cure certain ailments hundreds of years ago. Sastry and his team have elucidated the external production of gold nanoparticles through the utilization of the Fusarium oxysporum fungus and the actinomycete Thermomonospora sp. Furthermore, they have recorded the internal production of gold nanoparticles utilizing the fungus Verticillium sp.
According to research by Southam and Beveridge, bacterial cells that have been exposed to Au3+ ions may readily produce gold particles that are nanoscale in size. Under harsh biological circumstances, such as alkaline environments and slightly higher temperatures, alkalotolerant Rhodococcus sp. was employed to create monodisperse gold nanoparticles. Furthermore, filamentous cyanobacteria may produce globular, quadrate and octahedron gold nanostructures from Gold (I)-thiosulfate and Gold (III)-chloride complexes, and they have examined the mechanisms underlying this process. Nair and Pradeep documented the formation of nanoalloys and nanocrystals using Lactobacillus.
Silver Nanoparticles:
Silver nanoparticles are highly effective antiseptic agents against a variety of bacteria, including types that are highly resistant to antibiotics, for example methicillin-resistant Staphylococcus aureus. Klaus and his fellow researchers have revealed that the bacteria Pseudomonas stutzeri AG259, derived from a mine of silver, is proficient in reducing Ag+ ions within a concentrated aqueous solution of silver nitrate. The process of reduction outcomes in the creation of silver nanoparticles characterized by precise sizes and unique structures, predominantly formed in the bacterial periplasmic space.
Oxide Nanoparticles
An essential class of chemical nanoparticle produced by microorganisms is the oxide nanoparticle. These are viewed from two aspects: non-magnetic oxide particles and magnetic oxide nanoparticles.
Intracellular magnetic particles constructed of iron oxide, iron sulphides, or in cooperationare formed by magnetotactic bacteria. The co-precipitation approach was used to create magnetic Fe3O4 materials with a mesoporous structure utilising yeast cells as a prototype.
Other oxide nanoparticles, such as Titanium dioxide (TiO2), Antimony trioxide (Sb2O3), Silicon dioxide (SiO2), Barium titanate (BaTiO3), and Zirconium dioxide (ZrO2) nanoparticles, have also been researched.
Different Nanoparticles:
In biotic systems, a wide range of creatures use biopolymers like protein and microbial cells to create organic/inorganic composites with organised structures. There have been reports of microorganisms producing Lead carbonate (PbCO3), Cadmium carbonate (CdCO3), Strontium carbonate (SrCO3), Polyhydroxybutyrate (PHB), Zinc phosphateand Cadmium selenide nanoparticles.
The process of producing Zinc phosphate powders characterized by a butterfly-like micro-structure, with dimensions spanning from eighty to two hundred nm in length and ten to eighty nm in width. In accordance to Kumar et al., F. oxysporum may produce highly luminous CdSe quantum dots at room temperature.
FACTORS INFLUENCING MICROBIAL NANOPARTICLE SYNTHESIS:
Several factors influence the microbial synthesis of nanoparticles, including growth conditions, reaction time, metal ion concentration, temperature, pH and microbial strain.
The choice of microorganism plays a fundamental character in defining the nanoparticle properties. Gericke and Pinches discovered that two fungi cultures, V. luteoalbum and one more known as Isolate 6–3, were proficient in manufacturing gold nanoparticles inside their cells in a distinction of shapes and sizes. By manipulating factors such as pH, temperature, gold concentration, and the duration of subjection to AuC, its possible to some extent influence the pace of particle production and the size of the particles.
Environmental factors can affect the process of synthesis by influencing the microbial growth and metabolism. Optimization of these factors is crucial in order to achieve desired nanoparticle characteristics.
UTILIZATIONS OF NANOPARTICLES SYNTHESIZED BY MICROORGANISMS
The inimitable properties of microbial synthesized nanoparticles have directed to their utilization in diverse fields:
Medicine: Silver nanoparticles, synthesized by various bacteria and fungi, exhibit potent antimicrobic properties, making them valuable agents in wound healing and infection control. Gold nanoparticles are valuable in target specific drug delivery and imagery applications owing to their biocompatibility and convenient functionalizatio.
Environmental Remediation: Microbial synthesized nanoparticles offer a sustainable solution for contaminant removal from water and soil. Selenium nanoparticles produced by certain bacteria have been employed to remediate toxic heavy metals. Additionally, nanoparticles can catalyse degradation of pollutants through advanced oxidation processes.
Material Science: Microbial synthesized nanoparticles are finding their way into novel materials with enhanced properties. Incorporating nanoparticles into polymers improves mechanical strength and thermal stability. Nanoparticles also contribute to enhanced catalytic activity in energy conversion and storage devices.
Drug Delivery: Drug carriers consisting of nanoparticles have the ability to circumvent the blood-brain barricade and the tight epithelial intersections in the skin. This capability allows them to overcome the inherent obstacles that frequently impede the precise delivery of drugs to specific target regions. Bacterial strain MC-1, which contains magnetosomes, has been employed as a carter for drug delivery.
Antibacterial Agents: Silver nanoparticles, generated externally through the utilization of Fusarium oxysporum, can be amalgamated into fabric materials to mitigate or else lower the susceptibility to infections caused by morbific bacteria, for instance Staphylococcus aureus.
CHALLENGES AND FUTURE DIRECTIONS:
Despite of remarkable potential of microbial synthesis, certain challenges such as scalability, reproducibility and understanding the underlying mechanisms persists. It is prominent that juxtaposed to physical and chemical procedures, the creation of nanoparticles consuming microorganisms is a comparatively time-consuming procedure, often spanning several hours or even a few days. This biogenesis technique will develop considerably more alluring with a shorter time span for manufacture.
Monodispersity and particle size are two pivotal considerations when evaluating nanoparticle synthesis. Hence, it is imperative to thoroughly research operative supervision of particle size and monodispersity.
Advances in genetic engineering may enable tailored nanoparticle synthesis by modifying microbial metabolic pathways. Additional investigation is essential to unravel the intricate interplay between microbial physiology and nanoparticle formation.
CONCLUSION:
Nanomedicine is an emerging area of research that is rapidly growing and holds significant promise for improving the detection and management of human ailments. The biogenesis of nanoparticles by microorganisms is measured a hygienic, innocuous and ecologically approachable method, often referred to as “green chemistry” procedures. Microbial synthesis of nanoparticles presents a promising avenue for sustainable nanomaterial production with diverse applications. The intricate interplay between microorganisms and metal ions offers a fascinating area for scientific exploration. As our understanding of microbial synthesis deepens, we stand poised to unlock innovative solutions to pressing challenges in medicine, environment and material science.
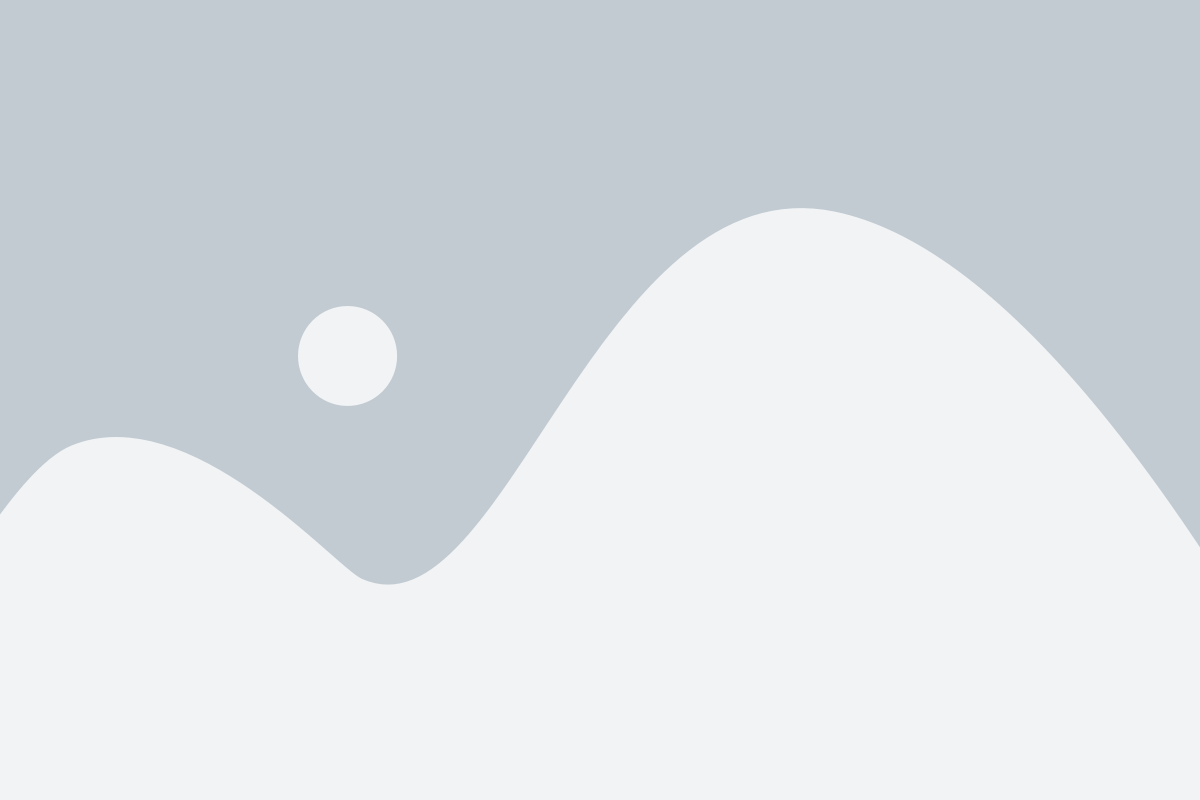
Dr. Yuvraj Yadav
Asst. Professor
School of Agricultural Studies
Related Posts
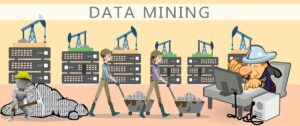
A Comprehensive Guide to Data Mining: Uncovering the Tricks to Locating Undiscovered Treasures.
A Comprehensive Guide to Data Mining: Uncovering the Tricks to Locating Undiscovered Treasures. The vast amount of information that surrounds us is subjugated by data. Every day, a vast amount of data is produced by both individuals and businesses, and
Sustainability and Green Engineering: A Path to a Greener Future
Introduction The rapid industrialization and technological advancements of the past century have led to remarkable progress but at a significant environmental cost. Issues such as climate change, resource depletion, and pollution are pressing concerns that demand immediate action. Sustainability and
Serverless Computing Optimization & Edge Functions
In the rapidly evolving world of cloud computing, serverless computing has emerged as a revolutionary approach to building and deploying applications. Unlike traditional computing models, where developers manage the infrastructure, serverless computing abstracts away the need for managing servers, allowing