Composite and Hybrid Structural Systems: A Comprehensive Guide.
UncategorizedComposite and Hybrid Structural Systems: A Comprehensive Guide
- January 9, 2025
- Geeta University
Ms. Feba S. Thomas
M.Tech(Structural Engineering)
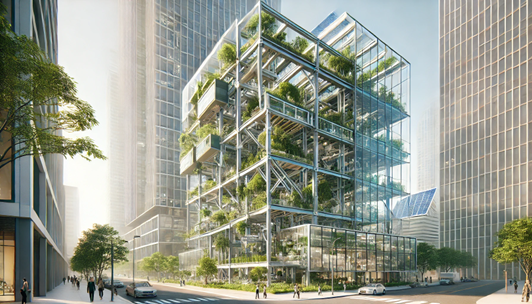
The demand for efficient, sustainable, and innovative construction solutions has pushed the boundaries of structural engineering. Composite and hybrid structural systems stand out as transformative approaches, integrating different materials or frameworks to maximize efficiency and performance. These systems have revolutionized modern construction by offering solutions to challenges such as load distribution, material optimization, and environmental sustainability. In this detailed guide, we explore these structural systems, examining their principles, advantages, applications, and challenges, while delving into the future of this exciting field.
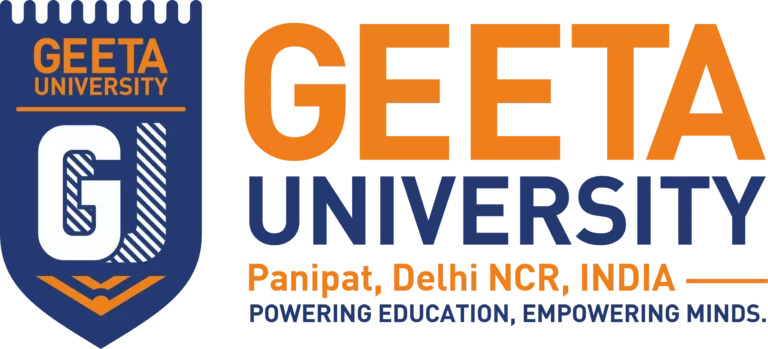
Admission Open 2024-2025
For Your bright Future
Composite Structural Systems: A Synthesis of Strength
What Are Composite Structural Systems?
Composite structural systems combine two or more different materials to form a single, unified structure that leverages the unique strengths of each component. These materials are bonded together in a way that allows them to share loads and stresses efficiently. Unlike traditional construction, where a single material often limits the structure’s capacity, composites capitalize on the synergetic interaction between materials. For example, a steel-concrete composite beam benefits from steel’s high tensile strength and concrete’s superior compressive strength.
The essence of composite systems lies in their ability to behave as a monolithic structure. Achieving this requires careful engineering, particularly in how materials are bonded. Techniques such as mechanical interlocks, shear connectors, and chemical adhesives ensure that the materials work in unison under applied loads, enhancing the overall performance of the structure.
Key Examples and Applications
- Steel-Concrete Composite Beams and Columns: These are staples in modern construction, used in high-rise buildings and bridges. The steel resists tension forces, while the concrete handles compressive stresses, creating a structure capable of supporting significant loads.
- Fiber-Reinforced Polymers (FRPs): FRPs are lightweight yet robust materials made by embedding fibers such as carbon, glass, or aramid in a polymer matrix. These composites are frequently used to strengthen concrete structures or as standalone elements in bridges and facades.
- Timber-Concrete Composites (TCCs): These systems combine the lightness and aesthetic appeal of timber with the durability and stiffness of concrete, making them an environmentally friendly solution for flooring systems in sustainable architecture.
The Science Behind Composite Systems
The performance of composite systems relies on understanding the materials’ properties and how they interact under different loading conditions. Steel and concrete, for example, expand at similar rates under temperature changes, ensuring compatibility in steel-concrete composites. Similarly, the orientation of fibers in FRPs can be tailored to resist specific stresses, such as bending or shear, making them highly adaptable to various engineering needs
Advantages of Composite Structural Systems
Enhanced Load-Bearing Capacity
One of the primary benefits of composite systems is their superior load-bearing capacity. By distributing stresses between materials with complementary properties, these systems can withstand higher loads and more complex stress patterns than traditional single-material structures. For instance, steel-concrete composite beams resist both bending and shear stresses efficiently, making them ideal for long-span constructions.
Material and Cost Efficiency
Composite systems allow for optimized use of materials, reducing waste and lowering costs. By using each material where it performs best, such as steel in tension zones and concrete in compression zones, engineers can achieve a balance between cost and performance. This efficiency is particularly advantageous in large-scale infrastructure projects, where even small savings per unit can translate into significant overall cost reductions.
Durability and Longevity
The integration of materials in composite systems often enhances their durability. For example, the concrete encasing steel in a composite column protects the steel from corrosion, significantly increasing the structure’s lifespan. Similarly, FRPs are resistant to environmental degradation, making them ideal for applications in harsh environments.
Hybrid Structural Systems: Combining Frameworks
What Are Hybrid Structural Systems?
Hybrid structural systems combine different structural frameworks or materials to create innovative solutions tailored to specific requirements. Unlike composite systems, where materials are bonded to act as a single unit, hybrid systems often feature discrete elements working in tandem. This approach allows designers to exploit the unique advantages of each system or material, achieving a level of flexibility and performance that would be impossible with conventional designs.
Hybrid systems can be classified into three main types:
- Structural System Hybrids: Integrate frameworks such as frames, trusses, and shell systems.
- Function-Based Hybrids: Use specialized elements for specific needs, such as vibration damping or thermal insulation.
Examples of Hybrid Systems
- Tall Buildings with Outrigger Systems: Hybrid systems incorporating outrigger trusses and core walls enhance stability by distributing lateral forces, allowing buildings to reach greater heights without compromising safety.
- Cable-Stayed Bridges with Reinforced Concrete Decks: These bridges blend the flexibility of cables with the rigidity of concrete, resulting in a structure that efficiently resists both dynamic and static loads.
- Timber-Steel Hybrid Frames: By combining timber’s sustainability and steel’s strength, these systems are increasingly popular in green building projects.
Advantages of Hybrid Structural Systems
Flexibility in Design and Functionality
Hybrid systems provide unmatched flexibility in addressing complex design challenges. By integrating multiple frameworks, engineers can adapt structures to meet unique functional requirements, such as optimizing seismic performance in earthquake-prone areas or incorporating renewable energy systems into the building envelope.
Enhanced Performance under Dynamic Loads
Hybrid systems excel in distributing dynamic loads, such as those caused by wind or seismic activity. For instance, tall buildings with hybrid systems can use tuned mass dampers or hybrid trusses to reduce oscillations, enhancing occupant comfort and structural safety.
Sustainability and Resource Optimization
By combining materials and systems intelligently, hybrid designs often result in more sustainable constructions. Locally sourced materials and prefabricated components reduce transportation emissions and construction waste, aligning hybrid systems with global sustainability goals.
Challenges and Considerations
Design Complexity
Both composite and hybrid systems require advanced engineering expertise and sophisticated design tools. Structural analysis must account for material compatibility, load-sharing mechanisms, and potential failure modes, making the design process more complex than traditional methods.
Construction and Maintenance
Construction of these systems often demands specialized equipment and skilled labor. Additionally, maintenance strategies must address the specific challenges of each material, such as protecting steel from corrosion in composite systems or monitoring joint integrity in hybrid frameworks.
Cost and Feasibility
While these systems offer long-term savings, their initial costs can be higher due to the need for specialized materials and technology. A thorough cost-benefit analysis is essential to ensure feasibility for each project.
Future Trends in Composite and Hybrid Structural Systems
As structural engineering evolves to meet the growing demands of modern construction, composite and hybrid structural systems are set to undergo significant advancements. Emerging technologies, sustainability initiatives, and the integration of smart systems are driving these changes. Below is a detailed exploration of the key future trends shaping these systems.
1. Integration of Smart Materials and Systems
The inclusion of smart materials into composite and hybrid structures represents a paradigm shift in structural engineering.
Shape-Memory Alloys (SMAs): These materials can return to a pre-defined shape after deformation, making them ideal for seismic applications. For instance, in hybrid systems, SMAs can be incorporated into joints or braces to allow structures to self-correct during and after seismic activity, reducing damage and downtime.
● Piezoelectric Materials: In hybrid systems, they can be used in sensors embedded within the structure to monitor real-time stresses, vibrations, or deformations. Such smart monitoring ensures timely maintenance and enhances the structure’s longevity.
● Self-Healing Composites: These advanced materials contain embedded capsules of healing agents (e.g., polymers or adhesives) that release upon cracking, automatically repairing the damage. These composites reduce maintenance needs and extend the life of structures.
The integration of smart systems with these materials is also noteworthy. For example, sensors embedded in hybrid frameworks can provide real-time data on environmental impacts, structural health, and energy efficiency, enabling adaptive responses to changes in load, temperature, or usage patterns.
2. Sustainable Innovations in Materials
Sustainability is a driving force behind modern construction trends, and composite and hybrid systems are no exception. Future developments are focusing on eco-friendly materials that reduce the environmental impact of construction without compromising performance.
● Bio-Based Composites: Materials such as bamboo, hemp, and flax are being used to reinforce concrete and polymers, offering an eco-friendly alternative to synthetic fibers like carbon or glass. Bamboo-reinforced concrete, for instance, provides a renewable and biodegradable reinforcement option that reduces carbon emissions during production.
● Recycled and Reclaimed Materials: The use of recycled steel, concrete, and plastics in composite systems is growing. These materials reduce construction waste and lower the carbon footprint of building projects. Hybrid systems utilizing reclaimed timber combined with advanced materials like FRPs are also being developed for sustainable architectural projects.
● Low-Carbon Cement and Geopolymers: Innovations in cement production are yielding low-carbon alternatives that can be incorporated into composite systems. Geopolymer concrete, made from industrial byproducts like fly ash, offers similar performance to traditional concrete with a significantly smaller carbon footprint.
These materials are particularly impactful in hybrid systems, where they can be selectively combined to optimize both environmental and structural performance.
3. Advanced Manufacturing Techniques
Modern manufacturing techniques are revolutionizing the way composite and hybrid systems are produced, making them more efficient, precise, and scalable.
● 3D Printing: Additive manufacturing is transforming construction by enabling the creation of complex composite structures with minimal material waste. For instance, large-scale 3D printers can produce FRP components with intricate geometries tailored to specific design requirements. These components are particularly useful in hybrid systems for custom joints and connectors.
● Modular Prefabrication: Prefabricated composite and hybrid elements are being manufactured off-site with advanced automation. This process not only reduces construction time but also ensures higher quality control. For example, hybrid frames combining timber and steel can be prefabricated with integrated systems like insulation and wiring, streamlining on-site assembly.
● Robotic Assembly: Robotics is playing an increasing role in constructing hybrid systems, particularly in assembling complex frameworks like steel-timber hybrids or cable-supported structures. This reduces human error, enhances safety, and accelerates construction timelines.
4. Adaptation to Climate Resilience
As climate change intensifies, the need for resilient structures is paramount. Composite and hybrid systems are being tailored to withstand extreme environmental conditions.
● Resilient Composites: New materials are being developed to resist harsh conditions such as high humidity, extreme temperatures, and salinity. FRPs with enhanced UV resistance or hybrid systems with corrosion-resistant coatings are becoming common in marine and desert environments.
● Climate-Adaptive Hybrid Systems: Hybrid designs are being optimized for adaptability. For instance, buildings in hurricane-prone regions are incorporating hybrid steel-timber frames with energy-absorbing connections that allow the structure to flex without failure during strong winds. In flood-prone areas, hybrid systems integrating buoyant elements can enable structures to float temporarily during high water levels.
● Energy-Efficient Structures: Hybrid systems are being integrated with renewable energy technologies such as solar panels, wind turbines, and energy-storing composites. For example, building facades with composite solar panels or hybrid bridges incorporating piezoelectric energy harvesters are paving the way for energy-neutral infrastructures.
5. Multi-Functional Hybrid Systems
Future hybrid systems are being designed to serve multiple functions, moving beyond structural integrity to incorporate features like thermal insulation, acoustic dampening, and energy generation.
● Thermal and Acoustic Insulation: Hybrid systems combining lightweight concrete, foam, and timber are being optimized for thermal performance, reducing energy consumption in buildings. Similarly, composites with sound-absorbing properties are being integrated into walls and ceilings to improve acoustics.
● Energy Storage and Generation: Some hybrid systems now include components that store or generate energy. For instance, concrete with embedded phase-change materials can store thermal energy, while steel-timber hybrid roofs integrated with solar panels provide on-site renewable energy.
6. Digital Twin Technology
The use of digital twin technology is rapidly expanding in the design and monitoring of composite and hybrid systems.
● Predictive Maintenance: By monitoring data from embedded sensors, digital twins can predict when a component in a composite or hybrid system might fail, allowing for proactive maintenance. This reduces downtime and extends the structure’s lifespan.
● Optimization of Performance: Digital twins enable engineers to simulate various scenarios, such as seismic events or wind loads, to optimize the design of hybrid systems. This capability ensures that the final structure is not only efficient but also resilient to unexpected stresses.
● Sustainability Tracking: Digital twins can track the carbon footprint of a structure during its lifecycle, providing insights into how hybrid systems can be optimized for environmental impact.
The future of composite and hybrid structural systems is brimming with possibilities.
The integration of smart materials, sustainable innovations, advanced manufacturing techniques, climate resilience, multi-functional capabilities, and digital technologies is redefining the potential of these systems. As these trends mature, they will enable the construction of structures that are not only stronger and more efficient but also adaptive, environmentally conscious, and aligned with the challenges of a rapidly changing world. By embracing these advancements, the construction industry is poised to shape a sustainable and resilient future.
Conclusion
Composite and hybrid structural systems represent the forefront of innovation in structural engineering. By combining materials and systems in ingenious ways, these approaches offer unmatched strength, efficiency, and sustainability. As the industry embraces new technologies and materials, the potential of these systems to transform the built environment continues to grow. From towering skyscrapers to sustainable homes, composite and hybrid systems are shaping a future where structures are not only more resilient and efficient but also environmentally conscious and adaptive to evolving challenges.
Tags
Related Posts
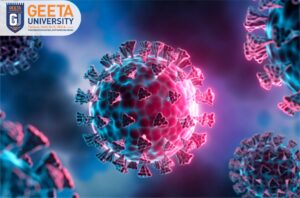
Education trends after Covid 19 – Geeta University
The COVID 19 pandemic flipped the world’s education system upside down this year, causing a significant shift in how pupils learn and teachers teach. Education Trends after Covid 19 The COVID 19 pandemic flipped the world’s education system upside down
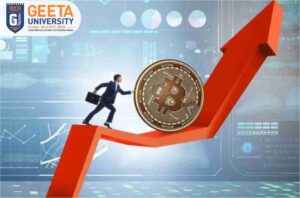
The future of Cryptocurrency in India – Geeta University
Gartner, leading research and consulting company, had predicted that the banking industry would receive $ 1 billion in business value in the use of blockchain Crypto currencies by 2022. The Future of Cryptocurrency in India Gartner, leading research and consulting
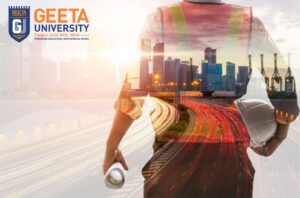
To be a Civil Engineer one must know – Geeta University
Civil engineering is in high demand for work. Working in this field requires very clear skills. It is not easy to find a great civil engineer and the best are richly rewarded. To Be a Civil Engineer one must know